By Shuhua Jin, Yiwei Dai, Nicholas Baruffi and Chihmin Cheng, Henkel Corporation
The use of medical wearables for self-health monitoring and digital care has risen rapidly in recent years. Most traditional medical light cure acrylate (LCA) adhesives contain isobornyl acrylate (IBOA), which has been reported to cause severe skin-sensitization in patients. To meet the increasing wearable market demands, more sustainable and skin-friendly LCA products are required. The developmental LCA resins are formulated to contain non-hazardous or non-skin-sensitizing monomers, providing a variety of viscosities and thixo ratios suitable for different dispensing methods. In this article, properties of two IBOA-free LCA resins, as well as an IBOA containing LCA resin, are reported. The effects of LED 405 nm curing intensity and curing time on polycarbonate adhesion strength on these LCA resins are studied using Design of Experiment (DOE). Solvent extractables of the cured resins will be presented in comparison with an IBOA containing one.
Introduction
Wearable technology is an electronic device worn on a user’s body. Wearable devices in healthcare are designed to collect data on users’ personal health and exercise. Examples of wearable devices in healthcare include wearable fitness trackers, smart health watches, wearable ECG monitors, biosensors, continuous glucose monitors (CGM) and blood pressure monitors, etc. Continuous health monitoring usually requires strong adhesives to connect the device covers to electronics or to attach the device to skin. However, the adhesives may cause patient skin sensitization and irritation, which are not acceptable to many patients. 1
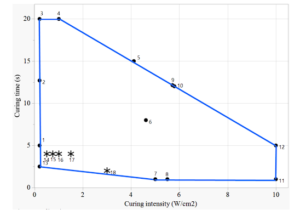
UV/visible light curable acrylate (LCA) adhesives for medical wearable devices offer various benefits. The light cure capabilities allow for quick and efficient bonding, reducing production time and increasing productivity. The adhesive also provides excellent adhesion to a wide range of substrates commonly used in medical devices and wearables, ensuring robust bonding and sealing performance after drop impact or bathing. Traditional medical LCA adhesives usually contain skin-sensitizing monomers as Isobornyl Acrylate (IBOA), which may cause patient skin reactions when used in wearables. IBOA has been reported in literature to result in severe allergic contact dermatitis due to its existence in the adhesive part of the sensor. 2-7 Many other skin-sensitizing acrylate monomers, such as 2‐hydroxyethyl methacrylate, ethylene glycol dimethacrylate and tetrahydrofurfuryl methacrylate, also were reported to cause a skin allergy. 8 With most of the acrylate monomers labeled as skin-sensitizing or skin-irritating in safety data sheets (SDS), it is becoming challenging to select safe monomers as part of wearable LCA compositions without compromising other properties.
Besides looking into a monomer’s SDS, the Primary Irritation Index (PII) is another way to evaluate its safety to skin. The PII of many acrylate monomers has been reported. In general, acrylate monomers with higher molecular weight and lower polarity tend to have lower potential for skin sensitization or irritation. Methacrylate has much lower PII than its acrylate analogues. 9 Alternative functional monomers, such as epoxy or thiol (if they can be cured in with acrylates and have a safer label), are good options for the wearable compositions as well. However, these substitutions or alternative options to make the product safer also will result in reactivity and property performance changes, which need to be considered and studied.
Many monomers, even labeled as skin-sensitizing or skin-irritating, have high photocurable reactivity and will be fully incorporated into the polymer network after proper curing. This presents less of an issue as no free monomer will migrate to skin surface. Therefore, it is important to choose the right curing parameters, especially the curing intensity and the irradiation time. This becomes more critical if the resin compositions contain oligomers and monomers with functionalities with different reactivities. The curing dynamics will affect the cross-linking and polymer network formation, leading to performance change, such as adhesion to substrates.
Whether higher curing intensity benefits the users is controversial. LED curing with high intensity up to 18 w/cm2 has allowed curing time to be significantly reduced, which means faster production for users. However, each adhesive behaves differently and may have its own limited maximum intensity. Beyond this limit, a higher intensity will cause an adverse effect. 10
In this paper, three experimental LCA formulations were studied and presented. Two formulas – LCA1 and LCA2 – are IBOA-free. One comparative formula – LCA3 – contains IBOA. LCA1 is an experimental formula and a derivative of the newly launched Loctite WT 3003 from Henkel, containing only non-hazardous monomers. It has no skin-sensitizing or skin-irritation statement in its US SDS. LCA2 is an experimental formula, a derivative of the newly launched Loctite WT 3001 from Henkel. It contains a skin-irritation monomer. LCA3 has similar composition as Loctite AA3926, containing IBOA and a skin-irritation monomer. The goal of this article is to compare the properties and extractables, as well as the effects of curing intensity and curing time on the bonding strength, of these three LCA resins.
Sample Preparation and Testing Methods
Materials
Three experimental LCA formulations – LCA1, LCA2 and LCA3 – were made and used for the studies. These materials contain oligomers, monomers, photoinitiators and other additives, such as fillers and stabilizers.
Curing
Samples were cured using Phoseon™ FireLine™ FL200 LED 405 nm or Henkel EQ CL30 LED Flood 405 nm. Curing intensity was measured by a Loctite® EQ PM20 UV/VIS radiometer 395-620 nm through the substrate.
Curing intensity and curing time DOE
Irregularly shaped Response Surface Design (RSM) DOE was used to design the two factors – curing intensity and curing time effect – on LCA1 sample. Curing time range is 1 s to 20 s; curing intensity range is 0.2 w/cm2 to 10 w/cm2. Linear constraints were used to eliminate curing conditions with too high curing dosage (high intensity and long curing time) and too low curing dosage (low intensity and short curing time). The design space is illustrated in Figure 1 with 13 DOE runs (Run #1-13 marked as dots) and five validation runs. Five additional data points (Run #14-18 marked as Asterisk *) were designed to validate the model. The response property is Polycarbonate to Polycarbonate (PC/PC) block shear strength. The DOE and data analysis were performed using software JMP 17.
Polycarbonate to Polycarbonate (PC/PC) block shear strength sample preparation and tests
PC/PC block shear strength was tested per ASTM D4501-01. The PC block substrate is UV-transparent medical-grade polycarbonate with dimensions of 25 mm x 25 mm x 6 mm. The substrates were cleaned with isopropyl alcohol before using. Adhesive was applied to create a bonding area of 25 x 12.7 mm with zero gap. The bonding area then was cured for the designed curing condition through the PC substrate using the Phoseon FireLine FL200. The block shear samples were tested at a crosshead speed of 0.08 inch/minute with five specimens in each group.
Extraction sample preparation and test
About 4 g to 5 g of light-curable composition was placed between two 6 x 8 mm empty space cuts from PE plastic sheets with a thickness of 0.75 mm and then cured for 20 seconds on each side using Henkel EQ CL30 LED Flood 405 nm with intensity of 1.0 w/cm2. A cured film was cut into strips of approximately 1 x 2 cm and placed in a 3-dram glass vial. Strips were added to the vial until the nominal aggregate weight was 2 grams. The vial was filled with 7 ml of acetone, and 250 µl of a decane internal standard solution was added. The vials were placed in an incubator and held at 38° C for 24 hours.
GC/MS analyses were conducted using an Agilent 7890B GC, 5977A MSD and 7650 ALS. The amount of an analyte extracted from a sample was calculated by Cx = (AxCis)/Ais)*(Vs/Wt.), where:
Cx = concentration of an analyte extracted from a film (ug/g)
Cis = concentration of the internal standard (ug/ml)
Ax = peak area of the analyte
Ais = peak area of the internal standard
Vs = volume of extraction solvent (ml)
Wt. = aggregate weight of cured film extracted (g)
Results and Discussions
Exp. IBOA-free resin property summary in comparison with IBOA containing resin
Table 1 is the property summary of the three experimental LCA resins: IBOA-free resins LCA1 and LCA2 and IBOA-containing resin LCA3.
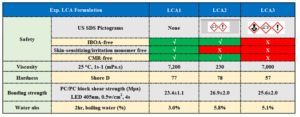
From a safety aspect in Table 1, LCA1 is classified as “Non-hazardous,” the “greenest” resin without any pictograms in its US SDS. LCA2 contains a skin-irritation monomer, but it’s IBOA-free and CMR- (Carcinogenic, Mutagenic, Reprotoxic) free. Comparative resin LCA3 contains IBOA, other skin-sensitizing and -irritating monomers, and CMR substances.
Both LCA1 and LCA3 are filled resins and have similar viscosities at a low shear rate. LCA2 has much lower viscosity. All three of the resins have similar PC/PC bonding strength under the specified curing conditions in the table. LCA1 and LCA2 have similar hardnesses, and LCA3 is a softer material. LCA1 has lower water absorption than LCA2 and LCA3.
DOE study on LCA1 with different curing intensities and curing times
Table 2 contains the DOE runs under various curing times and curing intensities, as designed by RSM DOE. Run order 1-13 are the DOE design spaces and are used for analysis and model building. Run order 14-18 are five validation points to validate the model. Response property PC/PC block shear strength was tested for each run and reported in Table 2. Curing dosage, the result of curing time multiplying curing intensity, also is listed for analysis.
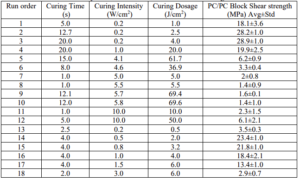
The results were analyzed using the standard least squares method. A model was established with adjusted R square of 0.88. Based on the Effect summary and PValue shown in Table 3, the main effects for the PC/PC block shear strength are curing intensity, quadratic of curing time and curing intensity, and quadratic of curing time and curing time.

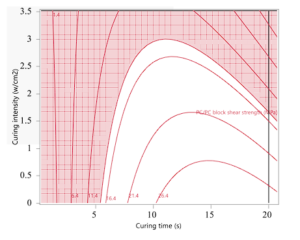
Figure 2 is the prediction contour profiler showing the PC/PC block shear strength values under different curing conditions. The value next to the red line in Figure 2 is the PC/PC block shear strength. If a PC/PC block shear strength greater than 15 MPa is set as the goal, all the white areas have the conditions meeting the goal, and the red areas don’t meet the goal. The contour plot indicates that the lower curing intensity and longer curing time favor the bonding strength. Curing time less than five seconds or curing intensity higher than 3 W/cm2 are in the red area, indicating that it is not able to achieve ≥15MPa bonding strength under these conditions. The prediction model has PRESS (Predicted error sum of squares) R-square of 0.82, which means the prediction accuracy is 82%.
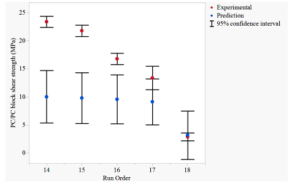
The model was further validated by five validation runs, Run order 14-18, in Table 2. The means of experimental results and the prediction results are labeled with red and blue, respectively, in Figure 3. The results in Figure 3 showed different prediction accuracy for different validation runs. For Runs 14-16, where the curing intensity is ≤1 W/cm2, there is no overlapping between the 95% confidence interval of experimental and predicted results. The actual bonding strength is higher than the predicated ones. For Runs 14-16, where the curing intensity is ≥1.5 W/cm2, more precise prediction can be provided by the model, and overlapping zones were observed.
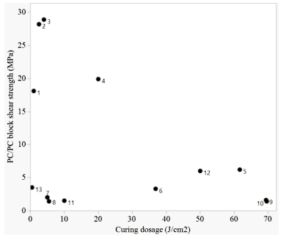
The correlation of PC/PC block shear strengths with curing dosage or curing energy based on the results in Table 2 also was studied. Figure 4 is the plot of PC/PC block shear strengths vs. curing dosage. High dosage commonly is considered beneficial to adhesive bonding strength, but some literature reported that an increase in exposure time increases bonding strength more than an increase in intensity. 11 In this study, no linear relation between curing dosage and bonding strength was observed. For this particular LCA1 resin system, the higher dosage resulting from high intensity has much lower bonding strength than the lower dosage resulting from low intensity.
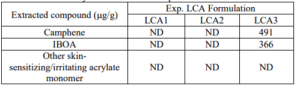
PC/PC block shear strength studies under three different curing intensities
From the above DOE study on LCA1 resin, it’s concluded that the curing intensity has significant effect on PC/PC bonding strength. This intensity effect on the other two resins LCA2 and LCA3 bonding strengths also were studied and summarized in Table 4. Three intensities 0.5, 1.0 and 1.5 w/cm2 were chosen based on the DOE results. The curing time for all the samples is four seconds. The failure mode of each sample also was reported. SF stands for Substrate Failure on PC, and AF stands for Adhesive Failure.
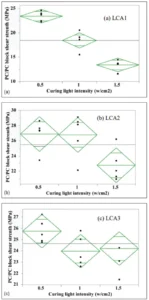
Figures 5 (a), (b) and (c) include the one-way analysis graphs of PC/PC block shear strength by curing intensity for resins LCA1, LCA2 and LCA3, respectively. Mean/ANOVA was used to analyze the statistical differences of PC/PC block shear strength by curing intensity for each resin. Editor’s Note: Analysis of Variance (ANOVA) is a statistical method used to test differences between two or more means.
The results showed that curing intensity affects the PC/PC block strength differently. For LCA1, there is a statistical difference of PC/PC block shear strength under the three intensities with p<0.05. For LCA2, there is no statistical difference between two intensities 0.5 and 1 w/cm2 (p>0.05), but there is a statistical difference between the intensities of 0.5 and 1 w/cm2 with 1.5 w/cm2 (p>0.05). For LCA3, there is no statistical difference of PC/PC block shear strength under all three intensities with p>0.05.
The results might be caused by the resin composition differences, including oligomer/monomer structures, functionalities, photoinitiator type and concentration, which lead to different “maximum intensities” 10 for different resins. LCA1 has the lowest maximum intensity, and LCA3 has the highest maximum intensity. When maximum intensity is exceeded, more polymerization cross-linking density, shrinkage and stress are possible, and some secondary reaction or outgassing may occur. These factors will cause cured-properties change and adhesion reduction.
Conclusion
In this article, the effects of LED 405 nm curing intensity and curing time on polycarbonate adhesion strength on experimental IBOA-free LCA resins were studied. For the LCA1 resin, in particular, it’s concluded that the curing intensity has significant effect on PC/PC bonding strength. Statistical difference varied with LCA2, dependent upon intensities, which may be caused by resin composition differences.
Acknowledgements
The authors would like to thank Jim Bernhardt for GCMS analysis of the extraction samples.
References
- K.R. Jinkins et al, “Thermally switchable, crystallizable oil and silicone composite adhesives for skin-interfaced wearable devices,” Science Advices, Vol.8, Issue 23.
- Y. Mine et al, “Allergic contact dermatitis caused by isobornyl acrylate when using the FreeStyle® Libre,” J Diabetes Investig. 2019, 10 (5): 1382–1384.
- Foti C, Romita P, Rigano L, et al, “Isobornyl acrylate: an impurity in alkyl glucosides.” Cutan Ocul Toxicol 2016; 35: 115–119.
- Busschots AM, Meuleman V, Poesen N, et al, “Contact allergy to components of glue in insulin pump infusion sets.” Contact Dermatitis 1995; 33: 205–206.
- Christoffers WA, Coenraads PJ, Schuttelaar ML, “Two decades of occupational (meth) acrylate patch test results and focus on isobornyl acrylate.” Contact Dermatitis 2013; 69: 86–92.
- Oppel E, Högg C, Summer B, et al, “Isobornyl acrylate contained in the insulin patch pump OmniPod as the course of severe allergic contact dermatitis.” Contact Dermatitis 2018; 79: 178–180. [PubMed] [Google Scholar]
- Raison‐Peyron N, Mowitz M, Bonardel N, et al, “Allergic contact dermatitis caused by isobornyl acrylate in OmniPod, an innovative tubeless insulin pump.” Contact Dermatitis 2018; 79: 76–80. [PubMed] [Google Scholar]
- Contact Dermatitis 1995; 33: 84–89.
- “Skin Damage Due to Acrylic or Epoxy Adhesives,” ThreeBond Technical News, Dec 25, 1992.
- Rainer Dörfler, Curing Adhesives: “When Less Intensity Is More,” Adhesive & Sealants, February 1, 2016.
- S. Yoshida et al, “Influence of light dose on bond strength of orthodontic light cured adhesives,” ResearchGate, April 2011, 34(4)493-7.