By Forough Zareanshahraki and Vijay Mannari, Coatings Research Institute, Eastern Michigan University
Editor’s Note: The following received RadTech 2018’s Best Paper Award in the Student division at the event held in May.
Abstract
Nail polish is one of the most widely used products in the US cosmetics industry, utilized by 117 million Americans in 2016, a number expected to reach 122 million by 2020. Gel nail polishes represent an advanced class of nail polishes, with the ability to cure under ultraviolet (UV) radiation, and consequently demonstrating improved properties and greater durability compared to conventional polishes.
Most gel nail polishes available today are based on petrochemical resources, making them unsustainable. Bio-based materials are excellent renewable resources with high potential of meeting final-product performance, cost and environmental needs. In addition, bio-based materials can be modified to make them amenable to cure by advanced UV LED exposure that consumes low energy and is safe for human exposure, compared to conventional UV mercury lamps. There has been a clearly growing consumer preference for use of products made from bio-based sources.
According to the US Department of Energy (DOE) technology roadmap, 10% of basic chemical building blocks should be derived from plant-based renewable resources by 2020, and this amount should increase to 50% by 2050. However, to the best of our knowledge, bio-based nail products have not been sufficiently explored. Therefore, considering the increasing consumption of nail polishes, and to keep pace with the bio-based regulations and consumer preferences, an unmet opportunity exists to develop novel sustainable nail gel polishes with considerable bio-renewable content.
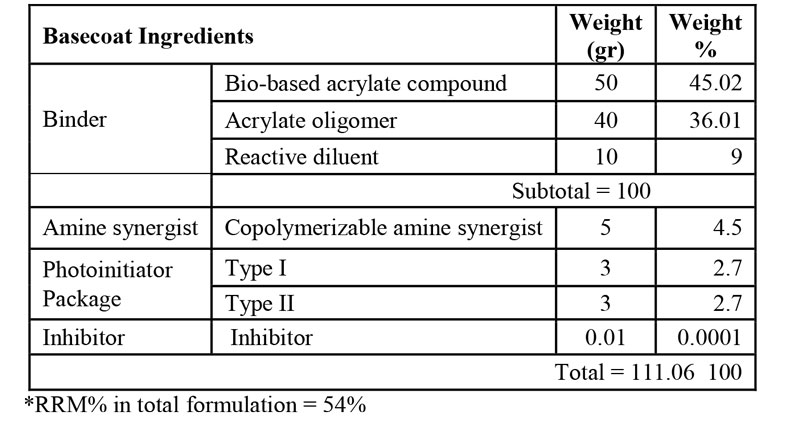
In this study, two green UV LED curable nail gel polish prototypes – one high-solid zero-VOC and the other waterborne, both with considerable bio-renewable content – have been designed and their performance compared with a commercial petro-based benchmark. Also, both formulations were cured under UV-mercury and UV LED radiation sources to evaluate curing efficiency. The high-solid formulation demonstrated promising performance, exceeding that of the benchmark, while the waterborne formulation met most of the desirable requirements, with some significant technical benefits. These novel nail gel polishes are greener alternatives to the current products in the market, with promising potential for consumer acceptance.
Introduction
Nail polishes are one of the most widely used products in the US cosmetics industry, utilized by 117 million Americans in 2016, with growth anticipated to reach 122 million by 2020. Nail lacquers are cosmetic coatings applied on human fingernails or toenails for two main reasons: first, for enhancing aesthetic aspects of the nails, and second, for protecting and strengthening fragile nail surfaces, serving as a protective cover for the nail tips 1,2. Conventional nail coatings usually contain various solid components, dissolved or suspended in a non-reactive solvent, which build a clear or pigmented film after application and drying on the nail. These nail polishes typically have low scratch resistance and will chip or peel from the nail surface after two to seven days in the best cases. Gel nail polishes are a specific class of nail polishes, with the ability to cross-link under UV radiation and, consequently, demonstrate improved properties and greater durability compared to the conventional polishes. Gel polishes typically last around two weeks without chipping or peeling3.
Gel nail polishes are usually offered in three layers:
- Base coat, which is applied directly on the nail, with different purposes such as covering the deformities on the nail surface and making it uniform, strengthening the nail and assisting with the adherence of the subsequent polish layer.
- Polish, as the colored layer, mainly provides aesthetic aspects.
- Clear topcoat is applied on the polish to form a hardened barrier, resistant to scratching, chipping and peeling. Each layer would be applied after curing the previous layer with UV radiation.
Although radiation curing offers unique features – such as fast cure and low volatile organic compound (VOC) emissions – in the development of nail gel polishes, health and safety aspects of using UV nail dryers has been a controversial subject for years. Although there are some observations that announce exposure to UV nail dryers – mostly radiating UVA – as a risk factor for skin cancer development4, in July 2017, the US Food and Drug Administration (FDA) released a statement about safety of UVA nail lamps5. The statement referred to a study by Dowdy and Sayre6 indicating that even for the worst-case lamp evaluated, 36 minutes of daily exposure was below the occupational exposure limits for UV radiation. (Note that these limits only apply to normal, healthy people and not to people who may have a condition that makes them more sensitive to UV radiation). Dowdy and Sayre reported that nail lamp outputs ranged from 0.5% to 2.2% of the allowable monthly occupational exposure limits – or 0.30 to 1.676 µW/cm2 between the range of 350 to 400 nm. As Diffey (2012) reports, this amount of risk is accepted by most women, and even that low risk could be virtually zero by using fingerless gloves at the time of curing the gel polish on the nails7.
Recent advances in Light-Emitting Diodes (LED) include high intensity of energy output and low initial cost. In addition, ability to through-cure, energy efficiency, longer lifetime and instant on/off ability have made UV LEDs an environmentally friendly technology with a lower carbon footprint compared to other UV curing technology.
“Bio-based materials refer to products that mainly consist of a substance(s) derived from living matter (biomass) and either occur naturally or are synthesized, or it may refer to products made by processes that use biomass.”8 These materials, such as plant oils, are excellent renewable resources with high potential of meeting final-product performance, cost and environmental benefits. In addition to this, bio-based materials can be modified to make them amenable to advanced UV LED curing. Thus, in recent years, there has been a growing interest for using them in the design and development of environmentally friendly advanced coatings9. With the US DOE technology roadmap goal of 10% of basic chemical building blocks being derived from plant-based renewable resources by 2020, and 50% by 205010, bio-based materials are leading the development of the next generation of products and processes. Thanks to their renewable agricultural and forestry base, bio-based materials are eco-efficient and generally economically viable.
The Renewable Raw Material content (RRM %) is one of the measures of product sustainability and is calculated according to Equation 1. However, although there are multiple studies on radiation-curable gel polishes, there is very limited focus on radiation-curable nail polishes based on renewable materials.
(Equation 1)
Review
Multiple researchers have patented radiation-curable nail coating formulations. Valenty et al.11 proposed a radiation-curable topcoat composition – composed mainly of nitrocellulose, (meth)acrylate monomers, nonreactive solvents, photoinitiator, inhibitor, etc. – to be applied on top of commercial nail enamels. They have reported that the proposed formulation was easily removable by standard acetone-based nail polish removers and is compatible with daily chores since it is water insoluble. Gudjil et al.12 designed a metamorphic radiation-curable nail polish consisting of a photochromic compound, such as Spiroxazine or Spiropyran derivatives, added to a clear polish comprising a base resin containing nitrocellulose, cellulose acetate butyrate and a photoreactive monomer that was able to react with UV radiation or sunlight by changing color from clear to any chosen color – such us violet, blue, yellow, red, etc. – and going back to its colorless form upon removal from the ultraviolet source. Cook et al.13 patented UV-curable nail coating formulations containing modified cellulose esters with ethylenically unsaturated pendant groups, acrylate monomers or oligomers as copolymerizable reactants, pigments, plasticizers, organic solvent, etc. The coating was formulated to be at least partially soluble in suitable removing solvents.
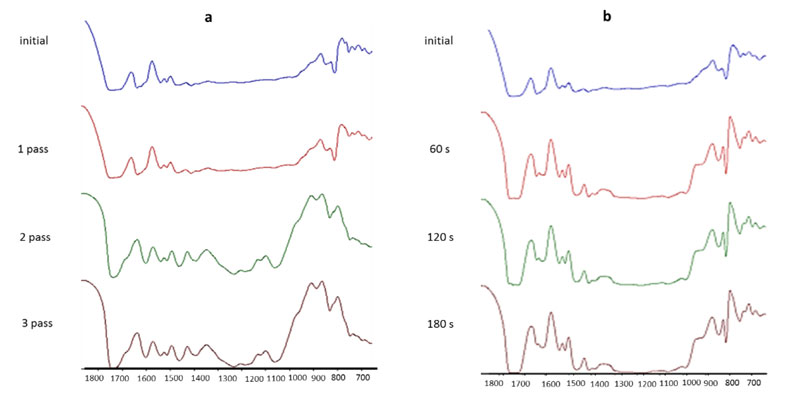

In another patent, Vu et al.14 proposed an easily removable base coat consisting of a 3D thermoset lattice dispersed in a network of solvent-dissolvable resin. The thermoset lattice provides durability, toughness and good adhesion, while the solvent-dissolvable resin facilitates removability. For making the 3D lattice, researchers used copolymers of polymethylmethacrylate and polymethacrylic acid, a solvent-sensitive monomer from the polypropylene/polybutylene glycol (meth)acrylate family, and other acrylate monomers such as urethane (meth)acrylates and cellulose esters were used as the solvent-dissolvable resin. As they reported when the polymer was exposed to a solvent, it penetrated to the domains of solvent-sensitive resin, dissolved it and then more easily penetrated to the interior of thermoset matrix. Proposing a storage-stable radiation-curable nail gel coating, Kozacheck et al.15 investigated effects of different organic and inorganic thixotropic agents on shelf life of pigmented nail gels consisting of urethane acrylate oligomers and (meth)acrylate monomers, reporting drastic differences in stability of the nail polishes (pigment settlement) with and without thixotropic agents. They also found out that by changing the rheological properties of the nail gels, thixotropic agents allow nail gels to be easily applied at lower viscosities due to shear thinning that reduces the amount of required solvent for viscosity adjustment.
Chang et al.1 patented a radiation-curable nail lacquer formulation mainly composed of aliphatic/aromatic urethane and polyester acrylate oligomers that contained no irritating reactive (meth)acrylate monomers, possessed good adhesiveness and was easily removable with a wooden or metal stick. Klang, Lu and Vappala16 disclosed UV-curable nail polish compositions based on aqueous polyurethane dispersions. They made a prepolymer using a diisocyanate compound, DMPA, a polyol and a compound containing both ethylenic unsaturation and hydroxyl. After neutralization, the prepolymer was chain-extended dispersed in water. Final nail compositions were prepared by addition of photoinitiator and – optionally – a leveling agent and a thickener.
Finally, Steffer et al. patented UV-curable nail coating formulations based on renewable polyols. These formulations consist mainly of a (meth)acrylate monomer or oligomer prepared from reacting the bio-based polyol with a (meth)acrylate monomer and a co-reactant, such as diisocyanate, polyacid, polyester, cyclic lactam, cyclic lactone, epoxy compounds, etc.17. To sum up, to the best of our knowledge, bio-based nail products have not been sufficiently explored by the researchers. Therefore, considering the increasing consumption of nail polishes and to keep pace with bio-based regulations and consumer preference, an unmet opportunity exists to develop novel sustainable nail gel polishes with considerable bio-renewable content.
By leveraging the environmental and health benefits of UV LED curing and bio-based oligomers/monomers, this study proposes new UV LED curable gel polish compositions with high bio-renewable content. In this effort, a zero-VOC high-solid (HS) UV LED curable nail gel formulation and a UV LED waterborne (WB) nail polish – both with considerable bio-renewable content – were designed and their performance compared with a petro-based product as benchmark. Each formulation was cured under both UV-mercury and UV LED radiation sources to evaluate the curing efficiency of the designed formulations by UV LED.
Experimental
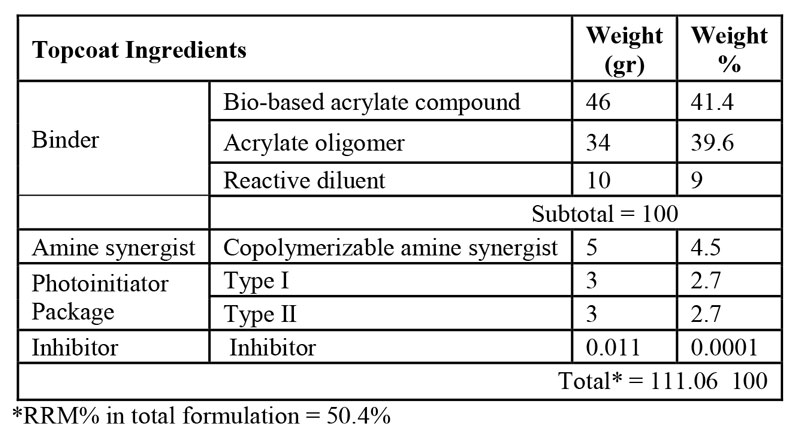
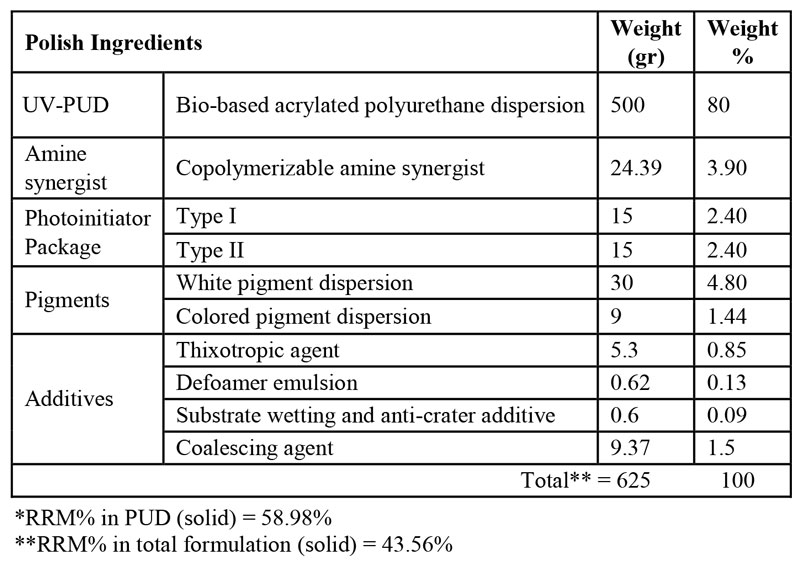
Materials
Aliphatic polyester polyol (Stepanpol pc-205P-160, Stepan), Bisphenol A epoxydiacrylate (Genomer 2252, Rahn USA Corp.), pigment dispersions both aqueous and in reactive diluents (Chromaflo Technologies), Garamite 1958 (BYK), Cellosize QP-300 (Dow Chemical), TEGO Foamex 822, Evonik), TEGO Twin 4200 Evonik, toluene diisocyanate (TDI, Byer), Genocure TPO-L (Rahn USA Corp) and isopropyl thioxanthone (ITX, BASF) were supplied by the manufacturers. Dimethylolpropionic acid (DMPA), acetone, N-Methyl-2-pyrrolidone (NMP), triethylamine (TEA), 4-methoxyphenol (MEHQ) and diethylene glycol diethyl ether were purchased from Sigma Aldrich. Mercapto-modified polyester acrylate resin (Ebecryl LED-02), hexafunctional aliphatic urethane acrylate oligomer (Ebecryl 1290), hexafunctional aromatic urethane acrylate oligomer (Ebecryl 220), Pentaerithritol triacrylate (PETA), copolymerizable amine synergist (Ebecryl P115), acrylated epoxidized-soybean oil (AESO, Ebecryl 860) and trimethylolpropane triacrylate (TMPTA) were provided by Allnex. Finally, isopropylideneglycerol methacrylate (Bisomer IPGMA) and a two-functional acrylate oligomer (Bomar BR-952) were supplied by GEO specialty chemicals and Dymax, respectively.
Synthesis of aqueous polyurethane dispersion for WB nail gel polish
DMPA, aliphatic polyester polyol, Bisphenol A epoxy diacrylate, acetone and NMP were charged into a three-neck flask equipped with agitator, nitrogen flushing tube, temperature controller and water-cooled condenser. The contents were heated to 80°C and held until the solution was homogeneous. Then, TDI was added drop-wise, and the batch was reheated to 80°C and held for one hour. After one hour, the temperature was increased to 90ºC and held for the % NCO target point. Percent NCO was determined by the di-n-butylamine back titration method, according to ASTM D2572. After that, PETA and AESO were added to the mixture to introduce acrylate functionality at the chain-ends. Reaction was continued until reaching to the NCO % near zero. Then, at 40 to 50°C, TEA was charged to the flask for neutralization and mixed for 5 to 10 minutes. Prepolymer was transferred to the dispersing vessels equipped with a high-speed dispersing agitator. Before dispersing the prepolymer in DI water, it was divided to three proportions. The first one was dispersed as it was; the second included 10% TMPTA, and the third included additions of 10% of the two functional acrylate oligomers. Agitator speed was increased to 1,000 to 1,500 rpm, and de-ionized water was added at a rate sufficient to maintain a vortex. After the complete addition of DI water, agitator speed was reduced to 300 to 400 rpm, and mixing continued for an additional 20 minutes. Finally, the PUD was filtered and transferred to a plastic container for storage.
Radiation curing
A SUNUV 48 W UV LED dryer machine with wavelengths in 365 and 405 nm and radiation intensity of 0.691 J/cm2for each 60s of radiation measured by a compact radiometer (UVPS) was used for curing the nail gel polishes. In addition, to evaluate the efficacy of UV LED curing of the designed formulations, a UV-mercury system (Fusion UV) with an H-bulb with the conveyor belt speed set to 12 ft./min. and energy density of ~0.70 J/cm2 per pass was also used.
All samples were applied with 2 mil wet film thickness on standard aluminum panels and were cured three times under 60s radiation period, or three passes under the UV mercury source at 12 ft./min. The WB formulation was first dried in the oven at 60°C for 10 min. to remove water before being polymerized under the UV LED or the UV-mercury lamp. The hardening and eventual full curing of the films were evaluated using a thumb twist procedure: The fully cured films did not leave any observable mark from placing a thumb on the film and twisting19.
Testing and evaluation
The following tests were performed in order to evaluate the bio-based nail gel polishes and compare their performance with the petro-based benchmark: Tack-free time, opacity, Acetone double rub, pendulum hardness test, pencil hardness. In addition, blush test (or water resistance) was evaluated by immersion of half coated plates in tap water for 4 hours, and then inspecting them visually after drying. Moreover, the removability of the nail gel polishes was assessed after 10 minutes of immersion in acetone13,14.
Furthermore, extent of cure vs. time for both curing methods was studied by time-based FTIR analysis using a Bruker Tensor 27 FTIR analyzer. Eight scans were recorded in the range of 400 to 4000 cm-1. Thin films of nail polishes were applied on prepared KBr pallets, and IR spectroscopy was performed after each pass of curing. To calculate the acrylate double bond conversion, the area of the acrylate band at 810 cm-1 was used. It was normalized using the carbonyl band (1720 cm-1), which is constant throughout the polymerization, as a reference peak. A comparison of the ratio of these areas for both the cured and the uncured sample allows calculation of the acrylate conversion degree after polymerization according to Equation 220. Finally, both HS and WB nail gel polishes were characterized for gloss at 60º using a micro-TRI Gardco gloss meter.
Result and discussion
Formulation design
Material selection
Several factors should generally be considered in material selection for developing nail gel polish formulations. First, the monomers and oligomers should not be selected from high skin-irritating acrylate monomers and oligomers. Second, the nail gel polish should be fast-cure under radiation, i.e., a tack-free film should be achieved in about one minute. Third, in order to prevent oxygen inhibition, which is highly probable in UV LED curing, acrylate content and reactivity should be controlled, since compositions with low reactivity or low acrylate content would be prone to oxygen inhibition. Oxygen inhibition could also be mitigated by using reactive chemicals, such as amines or copolymerizable amines, which contain easily abstractable hydrogen atoms18 and can react with proxy radicals. Copolymerizable amines are preferred in this study since acrylate functionality ensures that the materials become a part of backbone, reduce its odor and prevent amine bloom to the surface. Another requirement is that the gel polish should be easily removable by common polish removers. To meet the aforementioned requirements, each of the main components – including binder, photoinitiator(s), pigments and additives – should be carefully selected for both HS and WB formulations, as described in more detail in the following section.
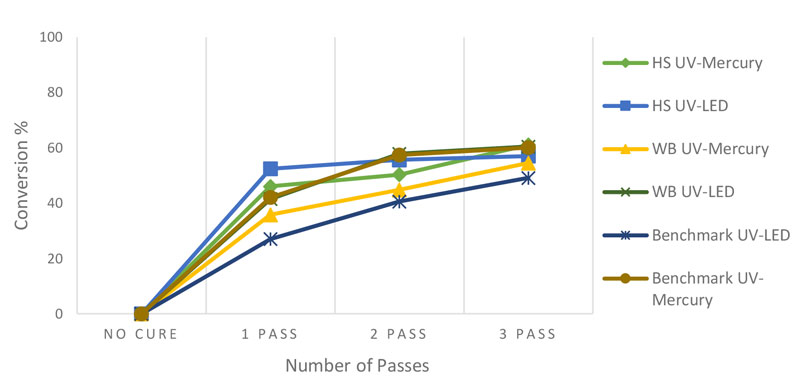

Binder. HS nail gel polish formulation. AESO and IPGMA were used as bio-renewable based oligomer and monomer, respectively. AESO, as a soft segment, also can contribute to easy removability of the nail gel polish. A mercapto-modified polyester acrylate was used to mitigate oxygen inhibition by increasing the cure speed. A hexafunctional aliphatic urethane acrylate oligomer and a hexafunctional aromatic urethane acrylate oligomer were used to provide desired acrylate content and also good chemical properties.
WB nail gel polish formulation. Regarding the WB binder, it should be noted that TDI and Bisphenol A epoxy acrylate were used in the PUD development to bring proper hardness, and AESO was used to bring bio-renewable content and acrylate functionality for UV curing.
Photoinitiators. UV LED lamps emit intense light in a narrow range of UVA and visible light wavelengths, therefore the number of useable photoinitiators is highly limited compared to those for conventional UV mercury platforms. Experiments have shown that using a combination of Type I and Type II photoinitiators can reduce oxygen inhibition more effectively18. After several preliminary experiments, a mixture of Type I, Type II and an amine synergist was found as the best choice for the initiator package in terms of faster curing (shorter tack-free time), lower oxygen inhibition, accepted level of yellowing and other properties, such as surface scratchability. Genocure TPO-L (Type I), ITX (Type II) and Ebecryl P115 (the synergist) were used as the initiator package in this study.
Pigment dispersion. To get an effective tint in the final coating, commercial pre-milled pigments in a monomer carrier, specially formulated for radiation-curable systems, were utilized. Among the available options, tripropylene glycol diacrylate (TPGDA) was chosen as the proper monomer carrier for pigment dispersion in this study. For similar reasons, aqueous pigment dispersions were used for the WB formulation.
Additives. In both formulations, MEHQ was used as an inhibitor to increase shelf life of the nail gel polishes. The amount of MEHQ was kept low to prevent poor cure and because of its effects on skin sensitivity. In the HS formulation, Garamite 1958 was used as a rheology modifier to prevent settling of pigments in long term. In the WB formulation, Cellosize QP-300 was used as the thixotropic agent. Other additives in the WB formulation include defoamer as well as anti-crater, wetting and coalescing agents.
Composition
As mentioned before, nail gels typically comprise three layers including base coat, color coat and topcoat. In this research, two three-layer bio-renewable based nail gel formulations were prepared and their performance was compared to a petro-based commercial three-layer nail gel polish as the benchmark. Tables 1 to 3 demonstrate the final composition of HS basecoat, polish and topcoat, respectively, and Table 4 demonstrates the formulation of WB nail gel polish. It should be noted that a small quantity of acetone, as a VOC-exempt solvent, was used in all formulations for dissolving ITX.
Cure study and performance properties
Although time-based Fourier-transform infrared spectroscopy (FTIR) studies were carried out for all the formulations, whole FTIR spectra are not included since they would occupy too much space for the purposes of this article. Thus, only the FTIR spectra for the HS formulation (Figure 1) will be represented here as an illustration. Figure 2 compares the extent of cure in WB and HS nail gel polishes by UV mercury and UV LED, as calculated by Equation 2. Based on these results, the extent of cure for each of HS and WB polishes is in a similar range in both curing methods. This shows that UV LED is an efficient curing method for the designed formulations. According to Figure 1, the majority of acrylic bond conversion occurs in the first pass of curing for both formulations, regardless of the curing method. In addition, in UV LED curing, the extent of cure is more than with the UV mercury lamp in the first and second passes for both formulations. In addition, in contrast to the waterborne formulation, the extent of cure in the HS polish by UV mercury exceeded that using the UV LED.

Table 5 demonstrates the results of evaluation of the commercial benchmark. Base coat, polish and topcoat were tacky after three passes (60s each) under the UV LED dryer, and the tackiness problem was not solved even after curing for 10 passes. Thus, after three passes and before the characterization, the very thin tacky layer was wiped off with a paper towel soaked with acetone, as is common in beauty salons, and is recommended in other studies14. The tackiness, most probably caused by oxygen inhibition, was not observed in the UV mercury curing method.
The HS designed nail gel polish layers, on the other hand (Table 6), became tack-free after one minute under the UV LED dryer, which is a considerably superior performance compared to the benchmark. As can be seen in the results, acetone double-rubs were in similar range for the layers, regardless of the curing method, which shows that curing was performed efficiently under UV LED exposure. However, the three layers demonstrated higher hardness when cured under UV mercury lamps, which might be caused by inevitable oxygen inhibition on the surface.
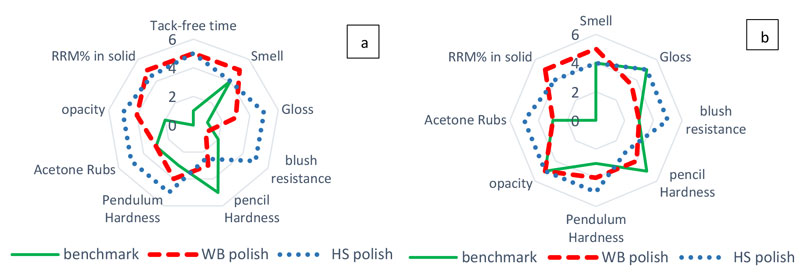
Waterborne nail polish was also completely tack-free after the first 60s of curing under UV LED. Table 7 summarizes the evaluation results of waterborne nail polish. According to the results, acetone double-rub is enhanced considerably by addition of acrylate monomer/oligomer, inducing more cross-link density. Hardness measurements were in a similar range, which shows oxygen inhibition is much less in the WB formulation. This is consistent with other studies that found less or no oxygen inhibition in aqueous systems because of lower solubility of oxygen in water compared to oil-based systems21.
All the HS layers – including base coat, polish and topcoat – passed the blush test, regardless of curing method. However, waterborne polish and benchmark layers failed this test and became hazy after immersion. Moreover, the addition of 10% TMPTA to the waterborne formulation improved water resistance drastically, which shows water resistance improves by increasing cross-link density.
As shown in Figure 3, LED-cured HS nail polish was glossy, showing 88.8% gloss at 60º. However, WB polish and benchmark polish were semi-gloss, showing 71.5% and 20.6% gloss at 60º, respectively. All formulations showed good surface adhesion but were easily removable from nail surfaces after 10 minutes of immersion in acetone. Figure 4 compares the overall performance of UV LED cured HS, WB and benchmark polishes and also the three-layer system.
Based on these results, HS Zero-VOC polish can be applied even as a single coat and meet the required properties for nail gels. In addition, WB formulation offers significant technical benefits, including low odor, the highest RRM % and the lowest oxygen inhibition. However, the waterborne nail gel polish needs to be applied as a three-layer system to demonstrate sufficient durability.
Conclusion
In this study, sustainable compositions containing more than 50 wt. % of bio-renewable materials were designed and developed using two different approaches: through a zero-VOC high-solid solventborne formulation and via a low-VOC waterborne formulation. The formulations were fine-tuned to rapidly and efficiently cure under UV LED radiation (as a safer source of radiation for human health and environment as compared to UV mercury sources) to obtain a completely tack-free surface after one to two minutes of radiation, using commercially available, low-cost UV LED systems. In addition, use of irritating (meth)acrylate monomers that could cause adverse allergic reactions was avoided in formulating the nail gels. Moreover, the nail gel polishes were easily removable after being soaked in acetone for a few minutes.


According to the evaluation results, the zero-VOC, high-solid formulation demonstrated promising performance, exceeding the petro-based commercial benchmark and waterborne samples in most of the required properties, and showed the possibility of application as a single-layer nail polish system. The waterborne formulation met most of the desirable requirements, with some significant technical benefits. These novel nail gel polishes are greener alternatives to the current products in the market with promising expectations of consumer acceptance.
References
- Chang, P.M.; Chang, C.H.; Chang, Y.C. Nail Lacquer Composition with Hyposensitivity. US20150190331 A1, Jul 9, 2015.
- Vu, T.; Conger, C.; Larsen, D. M.; Valia, D.; Schoon, D.D. Compositions and Methods for UV-Curable Cosmetic Nail Coatings. US8901199 B2, December 2, 2014.
- Klang, J.; Lu, J.; Vappala, I. Nail Polish Composition Based on Solvent-Free Aqueous Polyurethane Dispersions. WO2015165897 A1, November 5, 2015.
- MacFarlane, D.F.; Alonso, C.A. Occurrence of Nonmelanoma Skin Cancers on the Hands after UV Nail Light Exposure. Arch. Dermatol. 2009, 145 (4), 447–449.
- Consumer Updates – How to Safely Use Nail Care Products. https://www.fda.gov/ForConsumers/ConsumerUpdates/ucm563407.htm (Accessed Dec 4, 2017).
- Dowdy, J.C.; Sayre, R.M. Photobiological Safety Evaluation of UV Nail Lamps. Photochem. Photobiol. 2013, 89 (4), 961–967.
- Diffey, B.L. The Risk of Squamous Cell Carcinoma in Women from Exposure to UVA Lamps Used in Cosmetic Nail Treatment. Br. J. Dermatol. 2012, 167 (5), 1175–1178.
- Curran, M.A. Biobased Materials. In Kirk-Othmer Encyclopedia of Chemical Technology; John Wiley & Sons, Inc., 2000.
- Mannari, V.; Patel, C.; Li, W.; Kiamanesh, A. Soy-Based Building Blocks for Advanced Photocure Coating Systems. In Green Polymer Chemistry: Biobased Materials and Biocatalysis; ACS Symposium Series; American Chemical Society, 2015; Vol. 1192, pp 249–267.
- McLaren, J. The Technology Roadmap for Plant/Crop-Based Renewable Resources 2020; National Renewable Energy Lab., Golden, CO (US), 1999.
- Valenty, V.B. Quick-Drying Nail Coating Method and Composition. 5435994, July 1995.
- Goudjil, K. Metamorphic Nail Polish. US5730961 A, March 24, 1998.
- Cook, P.M. UV-Curable Nail Coating Formulations Containing Cellulose Esters with Ethylenically Unsaturated Pendant Groups. US5985951 A, November 16, 1999.
- Vu, T.; Conger, C.; Larsen, D.M.; Valia, D.; Schoon, D.D. Compositions and Methods for UV-Curable Cosmetic Nail Coatings. US8901199 B2, December 2, 2014.
- Kozacheck, J.E.; Lein, G.M. Easily Appliable, Storage Stable, Radiation-Curable, Pigmented, Artificial Nail Gel Coatings. US20140369944 A1, December 18, 2014.
- Klang, J.; Lu, J.; Vappala, I. Nail Polish Composition Based on Solvent-Free Aqueous Polyurethane Dispersions, 2015.
- Steffier, L.W. UV-Curable Nail Coating Formulations Based on Renewable Polyols. US8574558 B2, November 5, 2013.
- Arceneaux, J. A. Mitigation of Oxygen Inhibition in UV LED, UVA, and Low Intensity UV Cure. RadTech 2014.
- Green, W.A.; Sehnal, P.; Harper, K.; Anderson, D.G.; Husár; Liska, R; Griesser, M.; Rose, A.T. Novel Phosphine Oxide Photoinitiators; Rosemont, IL, 2014.
- Prandato, E.; Livi, S.; Melas, M.; Auclair, J.; Verney, V.; Fleury, E.; Méchin, F. Effect of Bio-Based Monomers on the Scratch Resistance of Acrylate Photopolymerizable Coatings. J. Polym. Sci. Part B Polym. Phys. 2015, 53 (5), 379–388.
- Ligon, S.C.; Husár, B.; Wutzel, H.; Holman, R.; Liska, R. Strategies to Reduce Oxygen Inhibition in Photoinduced Polymerization. Chem. Rev. 2014, 114 (1), 557–589