By Natasha Hausler Banke, Ph.D., INX International Ink Company
Editor’s Note: The following received RadTech 2018’s Best Paper Award in the Professional division at the event held in May.
Abstract
The current guidelines for the use of raw materials containing residual building-block chemicals, such as bisphenol A (BPA), vary by country and governmental agency, while the safety of these chemicals continues to be debated within the scientific community. The lack of agreement on safety and acceptability of these materials has created the need for ink manufacturers to eliminate the use of these raw materials completely and develop finished inks with alternative raw materials. Alternatively, we sought to develop a method to detect and quantify residual building-block chemicals in raw materials and finished printing inks. The current study developed a targeted method to detect and quantify residual chemicals in raw materials and ink formulations by high-pressure liquid chromatography (HPLC) tandem mass spectrometry (QqQ). This paper will present methodology and data on the detection of free BPA in raw materials and finished printing inks.
Overview
Bisphenol A (BPA) is a monomer used in epoxy, polycarbonate and corrosion-resistant unsaturated polyester-styrene resins1. BPA epoxy acrylate coatings provide a balance between performance and cost, offering rapid cure speeds, high gloss and hardness with good chemical, water and corrosion resistance. Interestingly, 80 to 90% of the BPA manufactured is used in nonfood-additive, nonmedical products, while only 5% is produced for use in food contact applications2. Epoxy oligomers used in “cure-in-place” technology offer low to zero volatile organic compound content along with improved performance and faster curing speeds. Cure-in-place formulations typically consist of a blend of an oligomer, a monofunctional monomer, a multifunctional monomer, a photoinitiator, additives and pigments. Diglycidyl ether bisphenol A epoxy acrylates are among the most commonly used oligomers in the UV curing industry.
The safety of BPA in food contact materials is controversial and widely debated. With suspected endocrine disrupting properties, concern regarding the suitability of BPA to be used in some consumer products has prompted review, research and much debate by the scientific community. The National Institutes of Health has funded numerous research grants and initiatives to evaluate the safety and bioactivity of BPA. With respect to BPA, the US Environmental Protection Agency is mainly focused on the general population exposure to BPA in drinking water and supports the United States Food and Drug Administration (FDA), as most human exposure “appears to come from food packaging.”2 With food packaging being the source of the majority of consumer exposure, agencies involved in regulating packaging also have studied the effects of BPA. The European Food Safety Authority’s (EFSA) Panel on Food Contact Materials, Enzymes, Flavourings and Processing Aids (CEF) evaluated and reviewed literature and studies on the toxicity of BPA at low doses and subsequently concluded the currently available data do not support that BPA has adverse effects on behavior3.
In Switzerland, the only country in Europe with legislation regulating food packaging, BPA falls under the Swiss Ordinance. As such, BPA-containing products can be used on food packaging but not in the production of polycarbonate bottles. The FDA has taken a similar stance to the use of BPA and has continued to support and fund research to evaluate BPA safety. In a recent statement released by the FDA, the initial review of a study conducted by the National Center for Toxicology Research (NCTR) core continues to “support [their] determination that currently authorized uses of BPA continue to be safe for consumers.”4 In the 2016 Nestlé Guidance Note on Packaging Inks, Nestlé took a more conservative approach by directing that no materials incorporating BPA in reacted form can be used on packaging5.

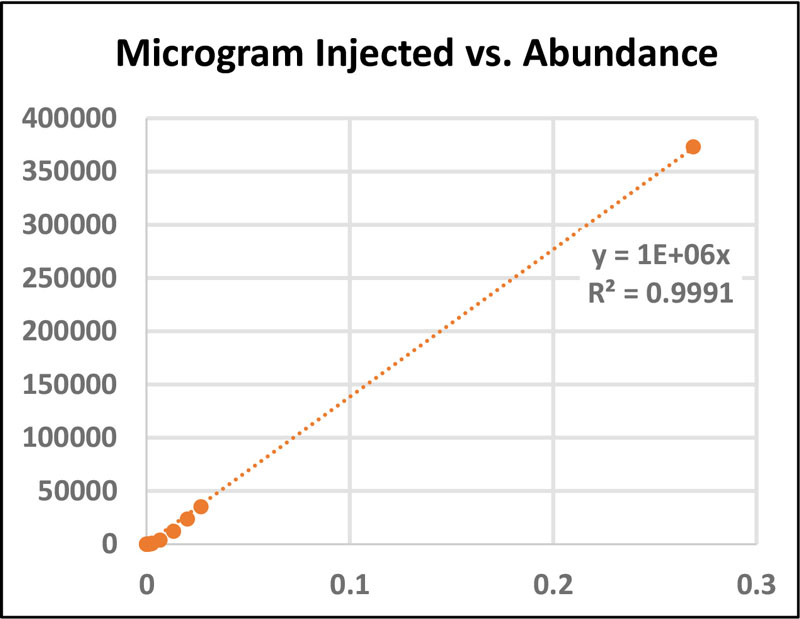
With little agreement on the safety of BPA among the scientific community and the absence of agreement on regulations among governing agencies, coating and ink manufacturers are left to either continue using BPA-containing materials or pursue alternatives. Alternatively, INX R&D Analytical pursued a risk assessment approach to using raw materials and finished inks that may contain residual BPA. In doing so, researchers developed a method to detect and quantify the presence of BPA in raw materials and inks that use BPA in the UV epoxy resin backbone. The two UV raw materials were chosen based on the vendor assertion that free BPA could be present in the raw material. One vendor asserted that while it does not regularly test for the presence of free BPA in its BPA epoxy oligomer resins, free BPA could be present in concentrations up to 5 ppm. The UV inks tested in this report were chosen because they contained one of the raw materials tested for the presence of free BPA. Once limits of detection and quantification are established, levels of BPA in inks and raw materials can be determined and correlated with total exposure to consumers.
Methods
A targeted, quantitative QqQ method on an Agilent 6460 was developed for detection and quantification of BPA. A certified reference standard from Sigma-Aldrich was purchased for detection optimization and instrument calibration. BPA has limited solubility in water and is readily soluble in organic solvents. With this in mind, all standard BPA solutions for detection optimization and calibration were prepared in Optima MS grade methanol. Targeted QqQ analysis uses a process called multiple reaction monitoring (MRM) to detect a parent, or molecular ion, then fragments the parent ion into daughter ions (m/z = 133 and 212) for detection (Figure 1). This process excludes analytes that are not of interest, while the MRM creates a fingerprint for positive identification and quantification of BPA. The detection of the transitions, 227→133 and 227→212, were optimized by flow injection analysis.
Due to the complexity of the ink samples and some raw materials, HPLC is required for the separation of sample components to accurately detect low levels of free BPA. Similar to previously reported methods, a reverse phase HPLC method was developed on an Agilent 1290 HPLC using a phenyl column. To elute BPA, the method utilizes a gradient of methanol to 1% formic acid in water with a reverse gradient back to original solvent mix. At the flow rate and solvent gradient, the retention time for BPA is highly reproducible and consistent at 3.6 min.
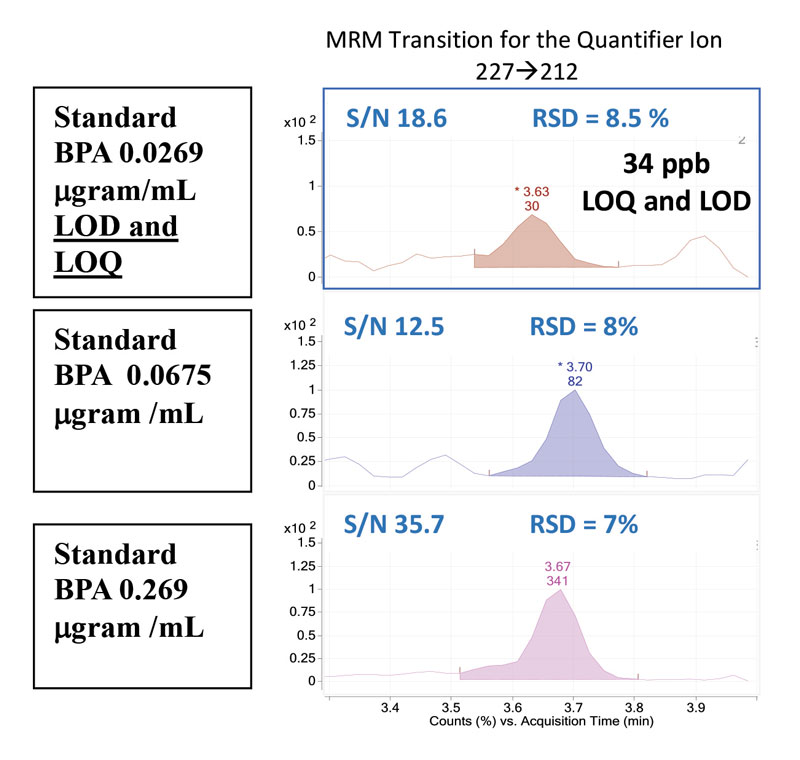
The optimized MRM detection method and HPLC gradient elution was used for developing the calibration curves and for detection of BPA in raw materials and ink samples. With the limited solubility of BPA, it is necessary to try several solvents to ensure the samples were completely dissolved for analysis. Methanol and water were chosen based on availability of solubility data and the HPLC solvent system. Acetonitrile was chosen for its effectiveness as an extraction solvent for BPA in several studies6-7. Lastly, methyl ethyl ketone was chosen for its reported properties to dissolve BPA and forming resins upon cooling8.

Results
A standard stock solution of BPA (0.0269 g/mL methanol) and serial dilutions (1:10) were prepared for flow injection analysis and to generate calibration curves (Figure 2). With the expected concentration of free BPA being less than 5ppm, intermediary dilutions were performed between lower concentration points to ensure linearity and accuracy.
When working with samples of very small content, such as building-block materials, calculating the threshold levels for detecting and quantifying BPA is imperative. The limit of detection (LOD) is the lowest analyte concentration which can be reliably distinguished from another analyte or the background, while the limit of quantification (LOQ) is the concentration that can confidently be quantified9. Both the LOD and LOQ are 34 ppb (Figure 3).
For determining the limits of quantification and detection, two parameters are evaluated: the signal-to-noise ratio and the relative standard deviation. Historically, the signal-to-noise (S/N) ratio is used to determine the LOD and LOQ, where the S/N = 3 is the LOD and the S/N = 10 signifies the LOQ10. Utilizing a targeted quantitation, where the signal from other compounds can be excluded or ignored, the calculated S/N can be artificially high. In this case, a statistical approach is employed to evaluate the precision of an instrument where multiple injections of a standard are made over several days. The relative standard deviation (%RSD = standard deviation/mean*100) is calculated. Instrument linearity is acceptable where %RSD = 10% and r2 = 0.9911. Additionally, accuracy is considered acceptable where the %RSD ‹ 20% for analyte recovery. For the current study, a conservative approach to determining the LOD and LOQ is applied, where both the S/N and %RSD are reported and evaluated.

Two UV-curable raw materials and UV-curable inks were tested for the presence of residual BPA. The raw materials and inks were analyzed in a similar manner as the standard BPA samples. BPA was not detected in the raw materials tested (Figure 4). The raw materials tested were not neat bisphenol epoxy acrylates. However, they are resins known to have residual BPA. In addition to testing raw materials, researchers tested finished inks for the presence of residual BPA. At the established LOD and LOQ, BPA was not detected in either of the inks tested (Figure 5).

When testing for residual chemical building blocks, researchers expect that the analyte content for residual chemicals will be at or below the limit of detection. If this is the case, the method must be validated by adding a known content of analyte, at three levels. This serves to test the method as well as test the detection of low analyte content. Due to the expected low concentration of BPA, all ink samples were spiked with a known amount of BPA with subsequent analysis (Table 1). The average spike recovery for ink A is 93% and 78% for Ink B with a percent relative standard deviation below 20%, for statistical relevance.
Conclusion and future implications
The current report presents a case for establishing and using analytical methods to regularly test raw materials and finished inks for residual building-block chemicals. By applying these methods for evaluating raw materials and finished inks, printing ink manufacturers will be able to make more informed decisions for UV ink formulations.
Nongovernmental organizations (NGO) and nonindustry entities are presently requesting “zero” levels of residual BPA. This requirement has far-reaching implications when using materials that are suspected to have low levels of BPA. In contrast, government agencies have set limits for BPA migration and exposure. For example, the Swiss Ordinance caps BPA migration at a specific migration limit (SML) = 0.6 mg/kg12, while the EFSA recognizes a tolerable daily intake (TDI) = 4 micrograms/kilogram body weight3. The EFSA estimates that the TDI is three to five times greater than actual BPA exposure3, while the RIVM recently reported that the TDI is likely 30 times greater than BPA exposure13. Presently, the FDA has not set a maximum exposure limit and cites the most recent NCTR report, which continues to support authorized uses of BPA being safe for consumers.
Due to the lack of agreement on the presence of BPA in raw materials and ink formulations, ink manufacturers are left in between NGOs and governmental agencies. In the context of detection of residual chemicals, setting the acceptable levels of BPA in a formulation equal to the limit of detection is a step to meeting both the criteria set by NGOs and governmental agencies.

Using the European Printing Ink Association (EuPIA) Good Manufacturing Practices (GMP): Printing Inks for Food Contact Materials guidance14 and the limit of detectable levels of BPA in cups and bottles set by the California Toxin-Free Infants and Toddlers Act (2011)15, the maximum amount of BPA in an ink can be calculated (Table 2). Where the maximum amount of BPA migrated into food is 0.1 ppb, the maximum allowable content in the ink (Cmax ink) of BPA is 416 ppb, which is well above the LOQ in the current method (Table 2). For comparison, a manufacturer of raw materials using BPA building blocks reports a maximum free BPA content of 5 ppm (5000 ppb) in several monomers. Where C max ink is 5000 ppb (5 ppm), Cmax food equals 1.2 ppb, more than 10 times greater than the amount set by the Toxin-Free Infants and Toddlers Act. Any formula using a raw material with 5 ppm free BPA cannot contain greater than 10% of that raw material, with the caveat that multiple raw materials with residual BPA would be additive.
By developing a method for BPA detection and applying it to detecting free BPA in raw materials and finished inks, we can begin to redefine what “zero content” means for nonintentionally added substances (NIAS). Currently, zero content means a compound is not present and not suspected to be present. However, by redefining zero and setting the Cmax ink equal to the LOQ, 34 ppb, the new achievable Cmax food is now well below the target limit of 0.1 ppb in a worst-case calculation. In this case, the residual building block in raw materials no longer limits the formulation, as in the case of a raw material containing 5 ppm BPA. By continuing to test raw materials for residual building-block materials and developing methods to detect the presence of other residual building-block materials (i.e. ortho-phthalates), ink manufacturers will no longer be limited to raw materials with “zero” content NIAS. By driving down residual content of NIAS, ink manufacturers will meet limits set by governmental agencies while satisfying NGOs zero content requirements.
References
- del Olmo, M.; Zafra, A.; Jurado, A.B.; Vilchez, J.L. Determination of bisphenol A (BPA) in the presence of phenol by first-derivative fluorescence following micro liquid-liquid extraction (MLLE), Talanta, 2000; 50:1141–1148.
- US Environmental Protection Agency, Bisphenol A Action Plan, 2010.
- European Food Safety Authority CEF Panel, Scientific Opinion: 2015 Risk Assessment, 2015.
- The United States Food and Drug Administration Statement from Stephen Ostroff, M.D., Deputy Commissioner for Foods and Veterinary Medicine, on National Toxicology program draft report on Bisphenol A, Press Announcement, February 23, 2018.
- Nestlé Guidance Note on Packaging Inks, 2016.
- Jordakova, I.; Dobias, J.; Voldrich, M.; Poustka, J. Determination of bisphenol A, bisphenol F, bisphenol A diglycidyl ether, and bisphenol F diglycidyl ether migrated from food cans using gas chromatography-mass spectrometry, Czech J. Food Sci., 2003; 3:85-90.
- Kang, J.H.; Kondo, F. Determination of bisphenol A in canned pet foods, Research in Vet. Sci., 2002; 73:177-182.
- US Patent, US3142688, 1964.
- Armbruster, D.A.; Pry, T. Limit of blank, limit of detection, and limit of quantitation. Clin. Biochem. Rev., 2008; 29:S49-S52.
- Dolan, J.W. The role of the signal-to-noise ratio in precision and accuracy, LCGC, 2006; 19:12–16.
- Ball, D.J.; Norwood, D.L.; Stults, C.L.M.; Nagao, L.M. (Eds), Leachables and Extractables Handbook: Safety evaluation, qualification and best practices applied to inhalation drug products. Hoboken, New Jersey: John Wiley and Sons, Inc. 2012: 435–438
- Annex 10 of the Ordinance of the Federal Department of Home Affairs (FDHA) on materials and articles intended to come into contact with food-stuffs, May 1, 2017.
- RIVM National Institute for Public Health and the Environment, The Netherlands, 2018.
- EuPIA Good Manufacturing Practice (GMP) Printing Inks for Food Contact Materials, 4th completely revised edition, 2016.
- California Toxin-Free Infants and Toddlers Act of 2011, effective date July 1, 2013