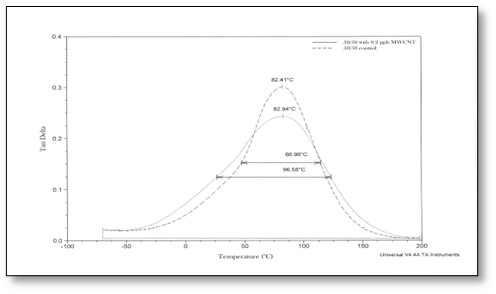
In four previous editions of UV+EB Technology, we discussed the preparation of nanocomposites made by incorporating carbon nanotubes (CNTs) into UV-polymerizable formulations. 1, 2, 3, 4 The first two of those articles summarized the experimental techniques developed by undergraduate students at the University of Houston-Downtown to determine how well single-walled carbon nanotubes (SWNTs) were exfoliated into an acrylate-functional medium and the effects observed of the SWNTs on the reactivity and other properties of UV-polymerizable systems. In that initial study, SWNT concentrations ranging from 0.00 to 0.20 pph based on the total mass of the monomers and the oligomer were evaluated.
The third article summarized studies of SWNT-containing UV-polymerizable formulations at concentrations from 0.35 to 1.00 pph of the monomer/oligomer mixture. That article also discussed the preparation of systems containing acrylate-functional urethane and polyester-based formulations containing multi-walled carbon nanotubes (MWNTs) at 0.20 pph. The fourth article in this series discussed the rheological properties of MWNT-containing formulations including “low shear” and slightly higher shear viscosity data. The purpose of Part 5 of this series is to summarize the thermomechanical (DMA) and thermal (TGA) properties of these MWNT-based formulations. Details of the experimental studies for this series of articles were reported at RadTech’s 2006 and 2008 UV/EB Conferences in Chicago, Illinois. 5, 6
Properties of UV-Polymerizable Formulations Containing MWNTs
SWNTs are carbon nanotubes containing single rolled graphene cylinders, while MWNTs are carbon nanotubes containing several concentric rolled graphene cylinders. The student researchers found, as described in Parts 3 and 4 of this series of articles, that using an updated dispersion technique involving enhanced sonication and stirring previously developed for SWNT nanocomposites produced MWNT-containing nanocomposites that were uniform in appearance and stable with respect to phase separation.
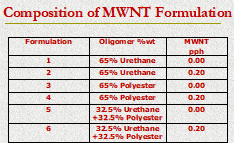
The MWNT-based formulations investigated in this study contained three different oligomer packages, all at 65%-by mass. The first formulation contained the same acrylate-functional urethane oligomer as in the SWNT study. The second one contained an acrylate-functional polyester oligomer, and the third one contained a 50/50-by mass mixture of the two. Table 1 shows the oligomers and MWNT compositions evaluated. Each formulation contained an equal mass mixture of the same three acrylate-functional monomers used in the SWNT study: isobornyl acrylate (IBOA), 1,6-hexanediol diacrylate (HDODA) and trimethylolpropane triacrylate (TMPTA). This monomer mixture represented 35.0%-by mass of the formulation.
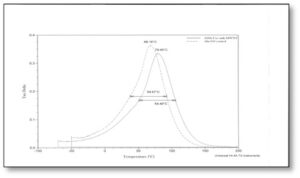
Thermomechanical Properties (DMA) of UV-Polymerizable Formulations Containing MWNTs
Polymers are known as “visco-elastic” materials. This means that they exhibit both “liquid-like” (visco) and “solid-like” (elastic) properties. Dynamic Mechanical Analysis (DMA) provides a means for determining both the liquid-like and solid-like properties simultaneously. How does this work? A polymer sample is subjected to a sinusoidal stress at a given frequency while ramping up the temperature at a constant rate. In a DMA experiment, the elastic properties are represented by the storage modulus curve and the viscous properties are represented by the loss modulus curve. The storage modulus curve indicates the ability of the polymer to absorb energy at different temperatures while the loss modulus curve reveals its ability to dissipate energy through frictional forces. The “tan δ” is the ratio of the loss to storage moduli at a given temperature and is useful in measuring the glass transition temperature (Tg) and the relative apparent homogeneity of the polymer film at the molecular scale. Figures 1-4 provide tan δ curves for the formulations tested in this study. The peak of each curve was taken to represent the Tg for each formulation and its half-height width was a measure of the inhomogeneity of the formulations; the relative level of nanogels in each sample. See Reference 7 for a discussion of “microgels” and “nanogels.”
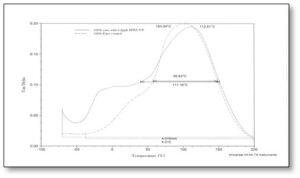
Figure 1 shows the tan δ curves for the polyurethane-based formulations (Formulations #1 and #2 in Table 1). Note from the peak of the two curves that the Tg has increased by approximately 11° C when MWNTs were included (solid curve). When relatively strong dipole-induced dipole attractions are assumed to be established between polar acrylate-functional monomers and oligomers, and the non-polar but very polarizable MWNTs, this is not surprising. But it, perhaps, is a bit more surprising that the half-height widths of the curves essentially are identical. That means that for the urethane-based formulation, the nanogel concentration did not appear to change upon addition of the MWNTs!
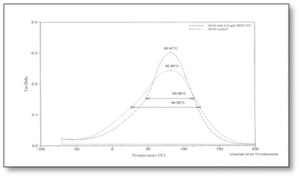
Figure 2 shows the tan δ curves for the polyester-based formulations (Formulations #3 and #4 in Table 1). The first thing to notice in these data is that the Tgs are significantly higher than for the urethane-based formulations; an average of about 34° C higher. This indicates that the acrylate-functional polyester oligomer may be significantly more polar than the urethane acrylate, creating stronger intermolecular attractive forces. Also notice that when MWNTs are added, the Tg increases by about 9-10° C, similar to the change occurring for the urethane-based formulations.
Perhaps the most significant difference observed between the urethane- and the polyester-based systems is in the heterogeneity of the polyester-based formulations. First of all, the half-height width of the polyester-based formulations is significantly larger than for the urethane-based formulations. Further, while the two urethane-based formulations appear to have essentially the same concentration of nanogels, the MWNT-containing polyester-based formulation has a profoundly higher concentration indicated. Not only is the half-height width larger, but also the MWNT-containing formulation has a substantial “shoulder” on the left side of the curve indicating an even larger number of nanogels than is indicated by the half-height width alone. Perhaps the heterogeneity of the MWNTs is being displayed in the case of the polyester-based formulations. But it is more likely that the MWNTs are in some way catalyzing the formation of additional nanogels when in the presence of the polyester acrylate oligomer.
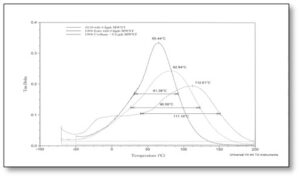
Figure 3 shows the tan δ curves for the 50/50-blend of the urethane- and polyester-acrylate oligomers with and without the MWNTs (Formulations #5 and #6 in Table 1). As might be expected, the Tg of this 50/50 system falls between that of the urethane-based and the polyester-based formulations. However, these data, ironically, indicate that the addition of MWNTs to the 50/50 formulation had no effect on the Tg, while having a significant effect (about 30° C) on the half-height width. This latter finding seems consistent with the results of the polyester-based formulation, although the very large “shoulder” present in the curve in Figure 2 substantially is reduced.
Figure 4 depicts an overlay of all three of the MWNT-containing systems demonstrating clearly the differences among them in Tg and nanogel concentration.
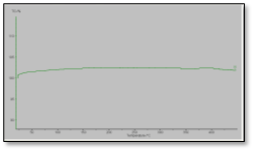
Thermal Properties (TGA) of UV-Polymerizable Formulations Containing MWNTs
In this investigation, the thermal properties of the formulations were determined using thermal gravimetric analysis (TGA). This analytical method involves measuring the temperature of decomposition of the samples and relates, therefore, to the thermal stability of the polymer. It is reasonable to assess the thermal stability of the MWNTs used in this study apart from the other formulation components. Figure 5 provides these results. It is obvious from the curve that the MWNTs have excellent thermal stability to temperatures above 500° C. Thus, any thermal breakdown at lower temperatures observed for composite materials can be assumed to be due to thermal instability of the polymer rather than the MWNTs.
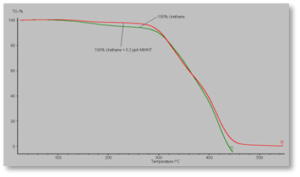
Figure 6 shows TGA data for the two acrylate-functional urethane-based formulations. The control formulation – represented by the green line – seems to have an initial thermal breakdown at a lower temperature than does the MWNT-containing formulation. However, this might indicate a small amount of volatile material, such as H2O (l), being driven off, particularly since the “decomposition” appears at near 100° C. More importantly, though, both the control and the composite formulation begin a nearly total breakdown at approximately the same temperature. This seems, again, to indicate that thermal decomposition is occurring within the polymer itself and not within the MWNTs.
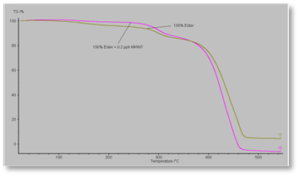
Figure 7 shows the TGA curves for the formulations containing the polyester acrylate oligomer. It shows similar behavior as seen with the acrylate-functional urethane oligomer. But note that the polyester-based formulations sustain only minimal decomposition up to about 380° C vs. about 280° C for the urethane-based formulation. Figure 8 shows a similar curve for the 50/50 blended oligomer formulation – except, in this case, the composite film and the control film essentially had identical thermal stability. The curves in this figure show the initial breakdown of the urethane- and polyester-based parts of the 50/50 blend to occur at essentially the same temperatures as in Figures 6 and 7.
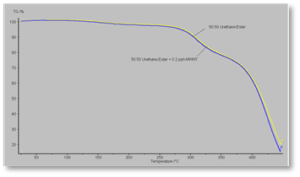
Figure 9 shows an overlay of all three composite films for direct side-by-side comparison. The order of thermal stability of the three formulations, then, is urethane-based < 50/50 oligomer blend < polyester-based.
Conclusions
This study gives strong support for the techniques developed for exfoliating SWNTs and MWNTs in UV-polymerizable formulations. Data have been presented that indicate an interesting and potentially useful set of rheological, thermomechanical (DMA) and thermal (TGA) properties of these nanocomposite materials. Ultimately, the polyester-based nanocomposites showed higher Tgs and thermal stability than the urethane-based formulations, while apparently producing a much higher concentration of nanogels. Hopefully, this work will stimulate interest among the readers of UV+EB Technology to conduct further research into this fascinating area of materials science.
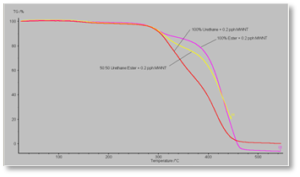
References
- “Professor’s Corner,” UV+EB Technology, Vol. 9, No. 3, pp. 16 & 17.
- “Professor’s Corner,” UV+EB Technology, Vol. 9, No. 4, pp. 14–16.
- “Professor’s Corner,” UV+EB Technology, Vol.10, No. 2, pp. 12 & 13.
- “Professor’s Corner,” UV+EB Technology, Vol.10, No. 3, pp. 14 & 15.
- V. Lam, G. Patino, C. Carandang, & B. Christmas, “UV-Polymerizable Systems Containing Single-Walled Carbon Nanotubes,” http://www.radtech.org/proceedings/2006/papers/093.pdf – Accessed August 16, 2024.
- N. Lam, D. Kurukji, S. Luce, & B. Christmas, “Acrylate-Functional Urethane- and Polyester-based Formulations Containing Multi-Walled Carbon Nanotubes,” https://www.radtech.org/proceedings/2008/papers/113.pdf – Accessed August 16, 2024.
- “Professor’s Corner,” UV+EB Technology, Vol. 6, No. 4, pp. 14 & 15.
Byron K. Christmas, Ph.D.
Professor of Chemistry, Emeritus
University of Houston-Downtown
b4christmas@gmail.com