By Peter E. Gordon
Bolb Corporation
Infectious diseases are caused by various pathogens: vegetated bacteria, bacterial spores, virions and fungi. Once transferred upon or introduced into a susceptible patient’s body, these pathogens, some of which are now antibiotic resistant, replicate and can cause an infection and illness, sometimes resulting in great discomfort and even death. Pathogens find their way to victims by way of contaminated food; suspect water; fomites, which are aerosolized pathogens in air or on dust; human contact with pathogen-contaminated surfaces; or human-to-human contact made during patient care. The preponderance and the associated danger of infectious pathogens in a health care delivery environment have been shown to be severely problematic. In 2011, 721,800 health care-associated infections (HAIs) were reported in the United States, with more than 7,500 deaths and an estimated cost to the health care system of $30 billion to $40 billion annually.1
The reduction of HAIs has subsequently become a top priority for health care facilities, with the highest emphasis placed on effective environmental hygiene. In US hospitals, $10 billion per year is spent on 1) hand hygiene, 2) decontamination of high-touch environmental surfaces and 3) sanitizing portable biomedical devices, data recording tablet computers and diagnostic equipment to sever pathogen transmission pathways. However, studies have shown that all three processes are often inadequate. For example, a study in 2008, found that 50% or more of high-touch surfaces may be routinely missed during daily cleaning.2
The improper choice or misuse of expensive, and – at times, toxic – cleaning chemicals can result in inadequate disinfection. Such errors leave environmental surfaces and equipment contaminated with pathogens, exposing patients and health care workers to pathogen transfer. Such carelessness greatly raises the risk of pathogen transmission to patients, visitors and health care workers along with the resulting manifestation of HAIs. Clearly, alternative approaches for achieving and maintaining the highest levels of environmental hygiene in hospitals are sorely needed. There is clearly an unmet need for effective methods of treating targeted surfaces that can be conveniently implemented to reduce dangerous pathogen loads.
Potential for germicidal light-based solutions
One method that has been gaining momentum is the episodic use, efficacy and convenience of germicidal ultraviolet (UVC) light applied to environmental cleaning of unoccupied hospital rooms and operating theaters. It has become more established in recent years, showing very promising results.3 However, the deployment of the assortment of no-touch ultraviolet disinfection systems on the market has acknowledged operational limits. For example, the systems only can be operated episodically in unoccupied space due to human safety requirements, and they are only validated for use on hard surfaces.4
As a result, fully taking advantage of the benefits of such high-powered germicidal-light surface disinfection delivery systems limits their wider deployment. Their use poses a particular challenge in high-traffic areas, fast-turnaround spaces and hard-to-reach surfaces, as well as with the potential use of untreated equipment due to unanticipated procedures being required.
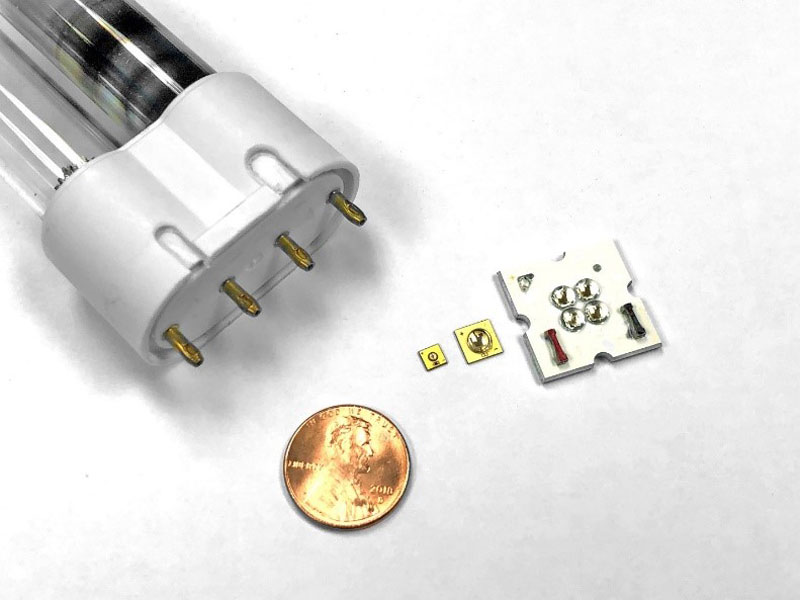
In response to this utilization gap, a series of published research raised the possibility of using germicidal visible light wavelengths for continuous sanitation of environmental surfaces during room occupancy. The most effective wavelength for this technique differs from the effective wavelength for UVC. It resides in the blue part of the visible spectrum, with peak activity at a wavelength of approximately 405 nm, and its use is being positioned as a means to cull the pathogen counts and help keep accessible hard surfaces cleaner between manual chemical treatments.5 It is claimed that absorption of 405 nm light by intracellular molecules induces production of reactive oxygen species, causing inactivation of pathogens. This kill method has been verified, and it has been acknowledged that the inactivation rate of 405 nm is much lower than that of UVC for the same applied dose. However, a much longer dwell time is required to achieve a meaningful degree of reduction.6,7
Given that the stated use of 405 nm light is during room occupancy – as an operational departure from use of UVC in an unoccupied space – it is vitally important that exposure to 405 nm has been demonstrated to be harmless to humans. Germicidal/405 nm light falls outside the unsafe-for-human-exposure UV wavelength band and, as a result, the US Occupational Safety and Health Administration (OSHA) does not consider 405 nm problematic and publishes no guidance on skin exposure limits. Published papers have demonstrated the safety and efficacy for use of 405 nm light to reduce acne vulgaris infections located deep within skin pores8, and the Food and Drug Administration (FDA) has issued 510(k) market clearance for medical treatment devices based upon these findings.9
These are clear indicators of skin safety, meaning that overhead light fixtures populated with 405 nm emitters can be used during room occupancy. However, it also is important to note that OSHA does set limits for eye exposure to 405 nm light, and any use of 405 nm light must be tested to conform to the other available international standards.10 After a trial safety and efficacy validation, one US hospital has implemented the approach in ceiling fixtures, attempting to suppress pathogen loads on operating theater and patient care room surfaces during health care worker and recovering patient occupancy.11
Each independent approach, episodic use of UVC or continuous use of 405 nm, has its time and place, benefits and tradeoffs12, entrenched supporters and detractors. Not surprisingly, there is no coordination or crossover of implementation, even though there should be, to the benefit of patients and hospital environmental service workers, and as a cost-containment approach. To the detriment of all such stakeholders, no bridge has yet been constructed.
However, one innovative approach, which holds great promise, has been suggested as a critical first span.13 It outlines the coupling of the established benefits of UVC between room occupancy episodic mode (UVC mode) with the burgeoning visible light during room occupancy continuous suppression mode (V mode). The objective is to more fully maximize germicidal light acceptance and greatly reduce pathogen loads on critical surfaces to the benefit of patients and hospital administrators alike. Such a dual mode system, with the ability to switch between modes, either by planned scheduling or by opportunity, offers high potential for enhanced efficacy and convenience. It would certainly result in a higher degree of surface sanitation on demand than is currently achieved, leading to more patient and worker protection as well as greater cost savings.
Use of Germicidal LEDs for a Dual Mode System
Germicidal light emitting diodes (GLED) represent a promising new source for pathogen inactivation in health care settings.14 Although LEDs emitting in the ultraviolet range have been only nominally explored for their implementation soundness and veracity of claims,15 with only cursory coverage given to their possible benefits,16GLED-based emitters – like their white light emitting counterparts – are small, robust, relatively low in cost, require no warm-up time and contain no toxic elements. In addition, GLEDs enable flexible mechanical design over rigid, straight, conventional low-pressure mercury germicidal lamps and their required bulky ballasts, allowing more flexible and configurable light delivery to specific target areas.
These attributes make them ideal light sources for the more mundane single wavelength solutions. Because they can additionally emit light at multiple individual wavelengths, providing fine tuning and selectivity, they are well suited for the envisioned, value-added, dual germicidal wavelength / dual mode surface treatment solution.
Problematically, the specific challenge with GLEDs is that they are still weak emitters compared to conventional germicidal lamps, so arrays must be placed very close to the target surface to produce high-surface intensity, which limits their positioning and coverage. But even with the current operational limits, GLEDs are being positioned to be the next wave in the LED lighting revolution. They present a new technological solution with enormous potential episodically for control of accumulated pathogen levels. As GLEDs emitting in the ultraviolet range (UVC LEDs), they continue to exhibit improved wall plug efficiencies, the case for deployment of such devices to address HAI reduction will become even more compelling.17
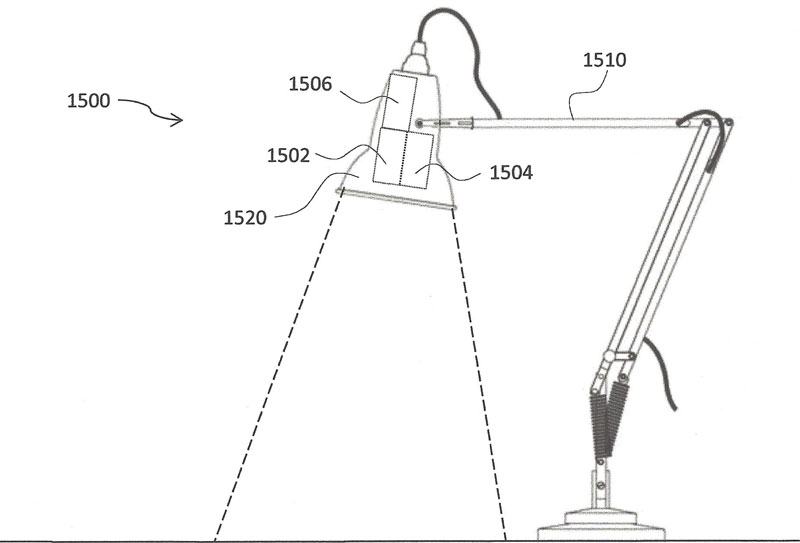
Studies have analyzed the response of different microbial strains to UVC LED irradiation, as well as the effectiveness of UVC LEDs with interleaving of LEDs emitting in the bactericidal UVC range from 255 and 280 nm.18 Similar studies concerned with food safety have been conducted with LEDs operating in the visible region (405 nm, 464 nm)19, and most relevant to the premise of combinatory dual-mode action, the effectiveness of using combinations of UVC and visible light germicidal lamps to inactivate pathogens can be traced back to a 1978 paper by Tyrrell and Peak.20 But only recently was there published follow-up work. It took advantage of the advent of arrays of GLED sources and the compelling indicator of the importance of sequential order on efficacy for extending the shelf life of cut produce.21
However, the specific, situational and operational advantages of a dual-mode approach in health care settings have yet to be verified. Such a solution combining 405 nm LEDs – for their safety yet weaker efficacy in a continuous pathogen suppression capacity (V mode) – with UVC LEDs – taking advantage of their greater efficacy but avoiding their inherent harmful effects on human vision and skin – for the heavy lifting episodic pathogen reduction mode (UVC mode) will be groundbreaking.
One possible configuration
The aim of the solution is the delivery of germicidal light emitted by LEDs to the entire surface area of various irregularly shaped objects that are specifically designated pathogen hotspots that are troublesome to keep clean in high-touch areas. The illumination would be achieved by unique use of targeted illuminating LED arrays of selected wavelengths situationally, depending on the nature of the work environment. One suggested design can be seen in Figure 2.
The range of the system may be up to 1 meter away from the dual emitters to cover larger targets – but not to be too great to avoid intruding on occupied space. The targeted inactivation areas illuminated by such a sanitizing system could be square, rectilinear, polygonal, circular or elliptical, and it may not necessarily be planar. For example, germicidal light could be guided to track the accessible area of a three-dimensional surface – such as patient care equipment, diagnostic equipment or chairs, tables and beds – as long as the uniform intensities requirements are met.22
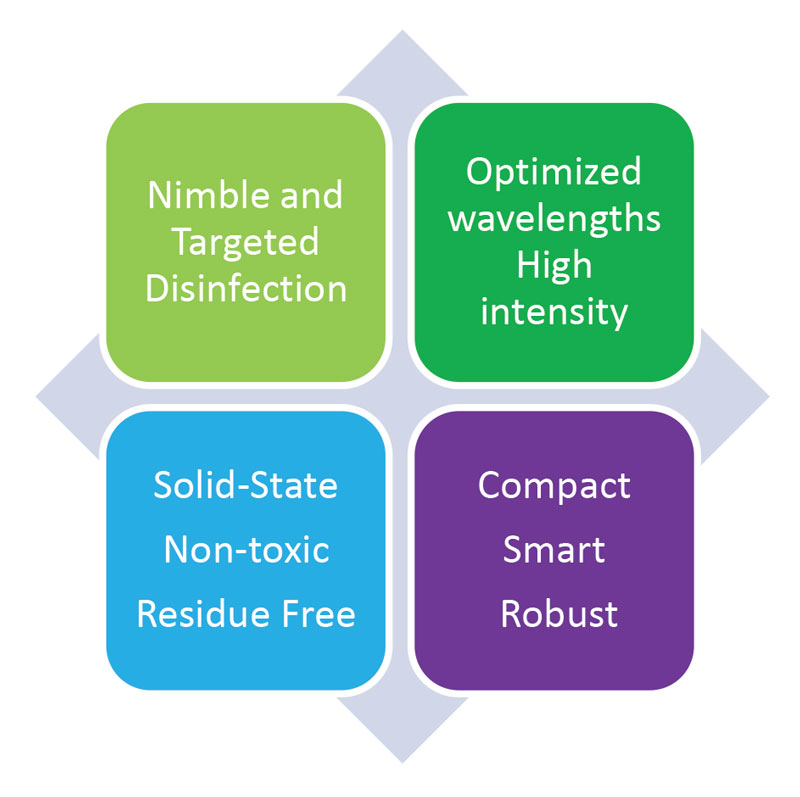
Similar to the well understood mechanics of germicidal UVC inactivation of pathogens, the effectiveness of the visible-light-emitting fixture in inactivating pathogens on a surface depends on the dose of germicidal light irradiating the surface. The number of pathogens inactivated by either UVC or visible light will vary with the irradiance and length of exposure, so rigorous efficacy testing – using the most up-to-date microbiological challenge practices – must be undertaken.23
Placement of the dual-wavelength emitter – coupled with a timed exposure generated by UVC LEDs and visible light, accomplishing repeatable, consistent and accurate surface sanitation each time – would introduce less restrictive operational requirements, safer work conditions for nurses, and overall greener and healthier facilities. The implementation is an ideal utilization, taking advantage of the attributes of GLEDs summarized in Figure 3.
Conclusion
The application of dual-operational mode, germicidal, LED-based decontamination systems to optimize surface sanitation of portable biomedical, data recording tablet computers and diagnostic equipment in acute care settings could help achieve the long-term goal of protecting recovering patients from exposure to infectious pathogens. Hence, it would improve patient outcomes by providing a safer environment of care, lowering the rates of HAIs.
Additionally, the deployment of such systems could further protect health care workers from exposure to the same set of infectious pathogens, providing a cleaner and safer work place during patient interaction. Such solutions would offer cost savings to hospital management seeking to decrease reliance on toxic antimicrobial agents and chemicals, along with the administration of increasingly pathogen-resistant antibiotics.
Resources:
- Centers for Disease Control and Prevention. Healthcare-associated Infections (HAI). 2015; http://www.cdc.gov/hai/.
- Carling PC. Evaluating the thoroughness of environmental cleaning in hospitals. The Journal of hospital infection. 2008;6(3):273-274.
- https://www.beckershospitalreview.com/quality/the-power-of-uvc-how-3-health-systems-slashed-their-hai-rates-through-a-hybrid-approach-to-infection-prevention.html
- https://www.xenex.com/video-demos
- M. Maclean, K. McKenzie, J.G. Anderson, G. Gettinby, S.J. MacGregor, 405 nm light technology for the inactivation of pathogens and its potential role for environmental disinfection and infection control, Journal of Hospital Infection 88 (2014).
- M. Hessling, B. Spellerberg, and K. Hoenes, Photoinactivation of bacteria by endogenous photosensitizers and exposure to visible light of different wavelengths – A review on existing data, FEMS Microbiology Letters, December 2, 2016.
- Rutala W A. et al. (2018). Antimicrobial activity of a continuous visible light disinfection system. Infection Control & Hospital Epidemiology 2018, 39,1250-1253.
- Gold, MH, Biron, JA, Sensing W. Clinical and usability study to determine the safety and efficacy of the Silk’n Blue Device for the treatment of mild to moderate inflammatory acne vulgaris. J. Cosmetic and Laser Therapy.2013.
- FDA 510K Predicate Devices include ATS-lI by TRIA Beauty, Inc. (formerly SpectraGenics, Inc.) (K060567) ThermaClear by Therative, Inc. (K060653) Zeno by Tyrell, Inc. (K043377) Tanda by Pharos Life Corporation (K080591).
- International Electrotechnical Commission – Photobiological safety of lamps and lamp systems, 2006. 2: International Electrotechnical Commission – Application of IEC 62471 for the assessment of blue light hazard to light sources and luminaires, 2014. 3: American Conference of Governmental Industrial Hygienists – Threshold Limit Values (TLVs) & Biological Exposure Indicies Signature Publications, Cincinnati, 2007. 4: International Commission on Non-Ionizing Radiation Protection – Guidelines on limits of exposure to optical radiation from 0.38 to 3.9 mm. Health Physics 73; 539-555; 1997. 5: International Commission on Non-Ionizing Radiation Protection – Guidelines on limits.
- Henderson Hospital becomes first hospital in the U.S. to install bacteria fighting operating room light fixtures. Indigo-Clean™ light fixtures installed to help keep patients safe at newly built hospital. November 1, 2016.
- http://sd.infectioncontroltoday.com/digital-issues/2016/10/uv-device.aspx
- Gordon, P. 2017, System and method for inactivating pathogens using visible and / or UV light. US Patent No. 080117.
- Messina, Gabriele et al., A new UV-LED device for automatic disinfection of stethoscope membranes, American Journal of Infection Control, Volume 43, Issue 10, e61 – e66.
- https://www.photonics.com/Articles/UVC_LEDs_Can_Combat_Hospital-Acquired_Infections/a62486
- UVC LEDs for Infection Prevention: Adding value to medical devices by integrating disinfection, CIS. July 2018.
- www.ledinside.com/products/2018/6/uvc_led_breakthrough_from_bolb_inc_to_drive_universal_disinfection_technology_adoption
- Beck, S. et al (2016) Evaluating UV-C LED disinfection performance and investigating potential dual-wavelength synergy. Water Research (109), 207-216.
- Motts, S. et al. The Use of 405nm and 464nm Blue Light to Inhibit Listeria monocytogenes in Ready-to-Eat (RTE) Meat. European Journal of Academic Essays 3(1): 76-80, 2016.
- Tyrrell, R. and Peak, M. Interactions between UV Radiation of Different Energies in the Inactivation of Bacteria. JOURNAL OF BACTERIOLOGY, Oct. 1978, p. 437-440. The paper reported a lethal interaction between various monochromatic wavelengths (254, 334, 365, and 405 nm) in the repair-proficient E. coli K-12 strain AB 1157, except in the case of pre-exposure to 405-nm which resulted in a protection against the inactivation resulting from subsequent exposure to 365- or 254-nm radiations.
- Gordon, P. UV-C LED for Food Safety. IUVA News / Vol. 20 No. 2, 4-7. 2018
- Gordon, P. 2017, System and method for inactivating pathogens using visible and / or UV light. US Patent No. 080117.
- ASTM E13135-18. Standard Practice for Determining Antimicrobial Efficacy of Ultraviolet Germicidal Irradiation against Microorganisms on Carriers with Simulated Soil. May 2018.
Peter E. Gordon is VP of Business Development for Bolb Corporation, Livermore, California, He is a 2018 recipient of a RadTech Emerging Technology Award and leads the IUVA “UV for Food Safety” Working Group. He holds an MS in Innovation Management and History of Technology from Edinburgh University, MSEE from the University of California, Santa Barbara, and a BSEE from the University of Pennsylvania.