By Jo Ann Arceneaux, Tong Wang and Hwei-Ling Yau, allnex USA Inc.; and Michel Tielemans, Kevin Poelmans and Laurence Boutreau, allnex Belgium SA/NV
Abstract
With the increasing regulatory pressure on acrylate monomers, the energy-curable graphics market has started to investigate the use of energy-curable waterborne resins and polyurethane dispersions (PUDs). Some products are particularly suitable for food contact applications, as illustrated by comprehensive migration tests and compliance with existing regulations. These energy-curable waterborne resins and PUDs also find utility in the development of next-generation low-viscosity inkjet inks and overprint varnishes where re-dispersibility in water is required. Data on the performance of these waterborne systems cured with both mercury lamps and LED lamps will be presented.
Inkjet ink technologies
Today, inkjet inks are either solventborne, waterborne or 100% solids energy-curable. Solventborne (SB) and waterborne (WB) inkjet inks are based on polymers, colorants and additives dissolved/dispersed in either solvent or water. These are well-known technologies, with a large installed equipment base. They are generally slow curing, with low resolution and color strength. The resistance properties of the cured inks also are rather poor. The solventborne inks have good adhesion to substrates, but due to volatile organic compounds (VOCs), are not environmentally friendly. Waterborne inks can have adhesion issues on nonporous substrates and also contain emulsifiers and coalescents, which contribute to VOC. Both of these technologies can dry in the print heads, causing blocked nozzles.1
The 100% solids energy-curable (EC) inkjet inks are very fast curing, with improved resolution and color strength. They have better resistance properties and are low in VOCs. However, some of the more opaque inks are difficult to cure because of film thickness. These EC inkjet inks are based on acrylated oligomers and monomers, pigments, additives and photoinitiators. They must contain high levels of acrylated monomers to reduce viscosity, and more of the monomer is mono-functional, due to the very low viscosity requirements. This monomer use results in odor and safety concerns, and in many cases, the Globally Harmonized System (GHS) labeling is not user friendly. The photoinitiator (PI) and its fragments also may create concerns for food packaging applications. [If electron beam (EB) cure is used, no PI is necessary, and this concern is eliminated.] Energy-curable inkjet inks do not dry in the print heads, so nozzle blockage is not a concern.2,3
Waterbased energy-curable inkjet inks combine the benefits of both waterbased and energy-curable inks and eliminate many of the drawbacks. These types of inkjet inks maintain the fast cure, good resolution, color strength and resistance properties of 100% solids EC inkjet inks and have low VOCs (no solvent, emulsifiers or coalescents). They also eliminate the use of low molecular weight monomers, resulting in lower odor and easing of regulatory and environmental concerns. The inkjet-printed product has lower dry film thickness, allowing better cure of opaque inks (but this still can be a problem) and providing a better hand or feel. This product is very durable and has better adhesion to plastics due to lower shrinkage. Waterbased energy-curable inkjet inks also separate the drying step from the curing step and are resoluble, so these types of inks do not block the nozzles. Thus, the value proposition for EC waterbased inkjet inks is 100% solids EC performance, with improved processability and VOCs over WB and SB inks, and improved safety over 100% solids EC inks.4
The EC waterbased inkjet ink value proposition opens the door to food packaging applications. Food packaging applications require global regulatory compliance [European Printing Ink Association (EuPIA), Nestle List, Swiss List, German Ordinance, US FDA REACH, Prop 65, etc.]; no use of chemicals of concern; no/low residuals, extractables or migrating species; no/low odor; and no/low VOC.5,6 The EC waterbased inkjet inks based on polyurethane dispersions (PUDs) can be designed to meet these requirements.
Design of energy-curable PUDs
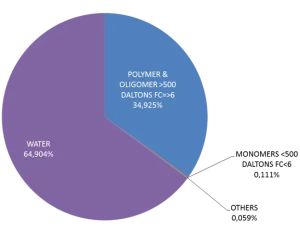
A cartoon of an energy-curable polyurethane dispersion (PUD) is shown in Figure 1. The composition of the EC PUD can be varied to provide for different properties. As shown in Table 1, EC PUDs can be designed to be label free (GHS) and bisphenol A (BPA) free. Tackiness and resolubility after dry but before cure are two properties that also can be controlled. Other parameters – such as migration potential, reactivity and flexibility – also can be varied. Although not shown in Figure 2, adhesion properties can be designed into the EC PUD.

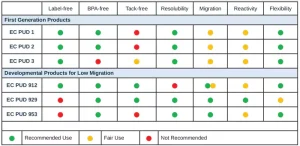
Waterbased energy-curable PUDs for food packaging
The composition of the EC PUD also can be designed to provide properties necessary for food packaging inkjet inks. Some of these properties are listed below:4
- Low viscosity without the use of solvent or monomers.
- High molecular weight to avoid extraction/migration; no low molecular weight (< 500) fractions. (However, EC PUDs are lower MW than traditional PUDs.)
- High functionality (>/= 6) to provide dense crosslinking and high performance.
- Controlled composition for other desirable properties such as stability, resolubility and adhesion.
The starting raw materials also must be controlled to avoid the use of chemicals of concern, such as tin, bisphenol A, alkyl phenol ethoxylates (APEO) and others. Figure 2 provides compositional data on EC PUD 929. This product contains greater than 99.5% (solids) of material with MW > 500 Daltons and functionality >/= 6, indicating its suitability for use in food packaging. (Mn = 1,875, Mw = 27,600 and Mw/Mn = 14.68.)
Validation of the suitability of the EC PUD for use in food packaging should include migration tests and identification of extractables. These tests were performed on EC PUD 929 and two reference EC PUDs that were not specifically designed for food packaging applications. A direct food contact protocol was used to address the most stringent extraction conditions. The polymer dispersions were formulated with a wetting agent (0 – 0.75%) and applied as a 30µ wet coating on an unwashed aluminum sheet. They were dried for four minutes at 60°C and then cured with an electron beam (250 keV – 5 MRad) with a conveyer speed of 10 m/min. The coated samples were immersed in 95% ethanol for 24 hours at 60°C in a closed glass flask. There was a contact area of 100 ml of solvent per dm2 of coated substrate. (These extraction conditions are related to FDA § 176.170 – conditions of use H, for paper and paperboard in contact with aqueous and fatty foods.)
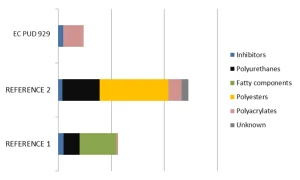
The alcoholic solution was submitted to GC-MS and LC-MS after collecting reference data from the pure polymer dispersions. Extractable components were identified and detected at suspected ppb levels by single ion monitoring (SIM). The MS response was recorded as peak areas for a comparative analysis, without considering that the MS response factors are different for each type of product. The extraction data from the two analytical techniques were merged and normalized so that they could be categorized per product type. As shown in Figure 3, EC PUD 929 shows very favorable migration data versus the non-food grade EC PUDs (shown as References 1 and 2). Based on these data, EC PUD 929 should be acceptable for indirect food packaging applications.
Energy-curable inkjet ink requirements
There are two major inkjet application techniques: continuous and drop-on-demand. Drop-on-demand is further divided into piezo and thermal (bubble jet) technologies. The 100% solids energy-curable inkjet inks are typically applied through piezo technology. Because of viscosity constraints, the 100% solids inkjet ink generally is heated to 40°C to 60°C before jetting. EC waterborne inkjet inks could be jetted by either piezo or thermal technologies.7
There are many requirements of an EC waterbased inkjet ink, and some of those follow. The inkjet ink must be stable, both during application and during storage. An elevated temperature stability test over a set period of time is the typical test (45°C to 60°C over various time periods). This accelerated aging test is used to predict room temperature shelf life of the inkjet ink. Viscosity, pH, particle size and/or coagulum are monitored during this test. The inkjet ink must be efficiently filterable to remove particles that may block or damage the inkjet head.
The inkjet ink must be jettable using whichever inkjet head is specified. Jettability typically is a function of surface tension, rheology and solids content. The inkjet ink must be resoluble before cure to avoid clogging the inkjet nozzles. Finally, the inkjet ink must be reactive or curable under the lamp and speed conditions of the specified press, and the cured ink must provide the adhesion and resistance properties required by the end application.8 These requirements will be further explained and discussed.
Ink stability
Figure 4 shows the results of a stability test of EC PUD 929 held for nine to 10 days at 60°C. The EC PUD 929 was diluted to 10% solids for this test. The particle size of EC PUD 929 decreased only slightly over nine days (80 nm to 75 nm). Over a 10-day period, there was a decrease in pH from 7.9 to 7.1 and in viscosity from 1.9 to 1.5 cP at 25°C. All of these results indicate a stable system. Cyan, magenta, yellow, black (CMYK) inkjet inks also were evaluated for stability over time at 50°C. Figure 5 shows the viscosity evolution in cP of these inks at zero, two, four and six weeks. Stability differences were seen with different EC PUDs and with different pigments, but good results were obtained for some inkjet inks.
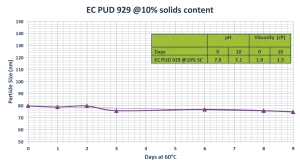
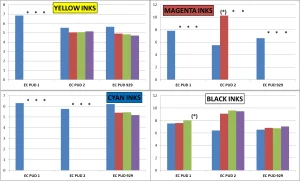
Ink filterability and jettability
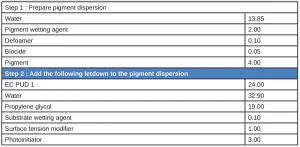
The EC PUDs and inkjet inks are filtered to remove particles that might damage or clog the nozzles. This must easily be accomplished without too much time and without excessive clogging of the filters. For ease in filtering the EC PUD, there is a specification on the amount of 50+ micron grits, typically < 10 mg/L. The inkjet ink must, obviously, be jettable. Filterability and jettability were tested on inkjet inks using the formulation shown in Table 2. Dimatix disposable piezo print heads were used in the jetting experiments. All CMYK inkjet inks could be filtered and jetted.
Ink resolubility
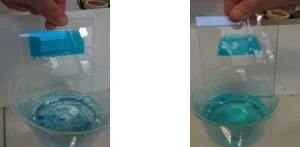
Ink resolubility before cure is important to avoid drying in the print heads and blocking the nozzles. As discussed earlier, inkjet inks based on EC PUDs separate the drying step from the curing step and are resoluble after dry but before cure. Thus, these inks do not block the nozzles. Figure 6 shows the resolubility after drying for several pigmented EC PUDs. [The dried films (on glass) were placed in water at room temperature for one minute.] All are resoluble, with EC PUD 929 a little less so.
Ink reactivity
Ink reactivity, or cure speed, can be affected by several variables. These include double-bond concentration of the ink, photoinitiator concentration, lamp type and output (wavelength and intensity), pigment color and concentration, and ink thickness, to name a few. With UV LED lamps, oxygen inhibition of the free radical polymerization also impacts surface cure speed.9
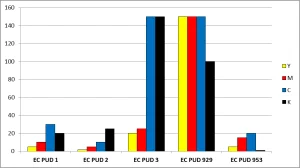
Figure 7 shows the surface cure speed in meters/minute for CMYK inks (proprietary formulations) based on five EC PUDs. (CMYK = Cyan, Magenta, Yellow, Black. The colors of the bar graphs correspond to the ink colors.) The inks were applied on Leneta charts at 15 microns (wet), dried in a 60°C oven for four minutes and cured using full spectrum mercury lamps (200 watts/cm at 70% power). Surface cure was measured via the graphite test for CMY inks and by the talc test for black (K) inks. EC PUD 929 had the fastest cure speed for all color inks. The EC PUD 929 has the lowest weight per double bond (172), but EC PUD 3 has the highest (602). So, it does not appear that weight per double bond is a major factor in determining cure speed.
UV LED cure speeds also were determined for clear coatings of EC PUDs. A #5 wire wound bar was used to apply the coating to a plastic substrate, which was dried for 10 minutes in a 60°C oven to yield a 5 micron coating. For curing, a 16 watt/cm2, 395nm UV LED lamp was used at a cure distance of 1 cm. Surface cure was measured via the graphite test.
The clear coatings were prepared by adding photoinitiator to the EC PUD and incorporating with a commercial mixer. Either ethyl (2,4,6-trimethylbenzoyl) phenyl phosphinate (TPO-L) or a water dispersion of phosphine oxide, phenyl bis (2,4,6-trimethyl benzoyl) (BAPO) was used at various concentrations.
Since EC PUD 929 was the fastest curing EC PUD with the mercury lamp (>150 m/min), it was chosen for the UV LED cure evaluations. With TPO-L as the photoinitiator at 1.5, 3.0 and 6.0% levels, surface cure speeds of 12 fpm (~5000 mJ/cm2) were obtained for all PI concentrations. Changing the PI to BAPO at 1.5, 3.0 and 6.6% levels resulted in uncured surfaces of the coatings when cured at 12 fpm. This is surprising, as BAPO has a higher extinction coefficient compared to TPO-L. However, BAPO was more difficult to incorporate versus the TPO-L, and this may have resulted in lower effective BAPO concentrations. (Even though the BAPO was water dispersed, a hazy product resulted after mixing with the EC PUD.)
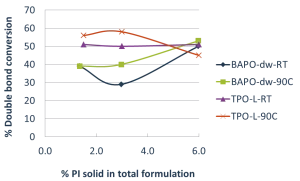
Figure 8 shows the double-bond conversion of the EC PUD 929 based clear coating with different PI levels and different cure temperatures. Conversion was measured by ATR-FTIR at 1.66 microns depth using the peak area at 810 cm-1 (C=C) and the reference peak area at 764 cm-1.10
Higher double-bond conversions were obtained with TPO-L versus BAPO, consistent with the cure speed results. (The exception was the 6% PI level cured at 90°C.) For room temperature cure, 1% TPO-L was as good as 3 or 6%. At 90°C, 3.0% TPO-L gave the best double-bond conversion. For BAPO, 6% levels gave the best double-bond conversion for both cure temperatures.
Increasing the cure temperature provides better mobility for free radicals in the coating and extends the time to reach the gel point. Both of these aspects will result in better double-bond conversion, as generally shown in Figure 8.11
Ink performance properties
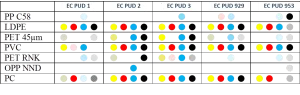
The cured inkjet ink must have adhesion to the substrate and provide the desired performance for the application. Table 3 shows the adhesion of CMYK inks based on EC PUDs to various substrates. The inks were applied on corona-treated filmic substrates at 15 microns (wet), dried in a 60°C oven for four minutes and cured using full spectrum mercury lamps (200 watts/cm at 70% power). Adhesion was tested by tape pull with TESA 4104 tape. The colors of the dots correspond to the C, M, Y or K ink, and the intensity of the dot reflects the amount of adhesion (darker dot = better adhesion). It was easier to obtain adhesion to some substrates (LDPE, PVC, PC), and some EC PUDs (2 and 3) provided better adhesion to multiple substrates.
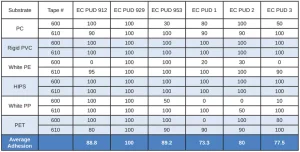
The adhesion of the clear coatings based on various EC PUDs and cured with UV LED is shown in Table 4. The coating (EC PUD and 3% TPO-L) was applied to the untreated filmic substrate with a #5 wire wound bar and dried for 10 minutes in a 60°C oven to yield a 5 micron coating. Cure was at room temperature at 12 fpm with a 16 watt/cm2, 395 nm UV LED lamp, at a distance of 1 cm (~ 5000 mJ/cm2). Adhesion was tested via tape pull (3M 600 and 610 tapes) and reported as percent coating that remained on the substrate.
With rigid PVC and HIPs, 100% adhesion was obtained for all EC PUD coatings. The coating based on EC PUD 929 had 100% adhesion to all of the substrates. EC PUD 912 and EC PUD 953 also were good for adhesion, with an average adhesion of just less than 90%. All EC PUDs achieved 100% adhesion to some substrates.
Generally, adhesion is related to through cure. These adhesion data show that through cure was achieved for all EC PUDs, even though surface cure was not. (Under these cure conditions, only EC PUD 929 achieved surface cure via the graphite test.) Cure with UV LED lamps generally provides better through cure than surface cure because of their longer wavelength emissions. These longer wavelengths are preferentially absorbed in the coating depth, and not at the surface, resulting in better depth of cure.9
Conclusions
Waterbased EC inkjet inks are an alternative to both waterborne inkjet inks and 100% solids EC inkjet inks and bring multiple benefits over the other technologies. EC PUDs can be designed to provide low migration and low extractables, and this provides the potential to formulate waterbased EC inkjet inks and coatings for food packaging.
Inkjet inks based on EC PUDs have been shown to be thermally stable, filterable and jettable. These inks also are resoluble after dry but before cure, ensuring that nozzle blockage does not occur. Reactivity of waterbased inkjet inks cured with full spectrum mercury lamps is dependent on the choice of the EC PUD, but can be quite fast. These inkjet inks also have good adhesion to many corona-treated filmic substrates. UV LED surface cure speed of EC PUD clear coatings needs improvement, but through cure is quite good, providing excellent adhesion to multiple untreated filmic substrates. υ
References
- Handbook of Industrial Inkjet Printing, Volume I, pp 27-28. W. Zapka, Ed. Wiley-VCH 2018.
- Ibid., pp 129-138.
- Ibid., pp 59-111.
- Ibid., pp 48-56.
- Ibid., pp 108-111.
- Ibid., pp 142-148.
- Ibid., pp 313-319.
- Ibid., pp 33-37.
- Ibid., pp 117-127.
- Chemistry & Technology of UV & EB Formulation for Coatings, Inks & Paints, Volume I, pp 245-250. R. Mehnert, A. Pincus, I. Janorsky, R. Stowe, and A. Berejka. John Wiley & Sons 1998.
- Ibid., pp 209-214.
Surface-cure test methods: Graphite and Talc Tests
These are surface cure tests. The graphite or talc is sprinkled on the surface of the cured ink or coating and then gently brushed off with a cotton ball. The amount of graphite or talc that remains is visually assessed. The visual assessment is made easier by using the graphite test on clear coatings and CMY inks, and the talc test on black inks.
Acknowledgements
My co-authors for all of the experimental results that were presented in this paper.