Food packaging and flooring, shrink-wrap and sterilization, cold seal adhesives and so much more – electron beam (EB) is a versatile technology used in a multitude of applications with potential yet untapped.1-3 The key reason for this versatility is electron beam’s ability to break chemical bonds without the assistance of an initiator and under ambient conditions. While EB is not unique in this ability (other technologies include x-ray and gamma ray), it certainly is distinctive when compared to similar applications of thermal and UV technologies.
Broken chemical bonds produce free radicals (unpaired valence electrons), which drive the free-radical chemical reactions that make up the vast majority of current industrial EB uses.1 These reactions include cross-linking, chain scission, polymerization and grafting. The diversity of reactions an electron beam can initiate, simply by exposing a material to accelerated electrons, has propelled the technology into a variety of markets.
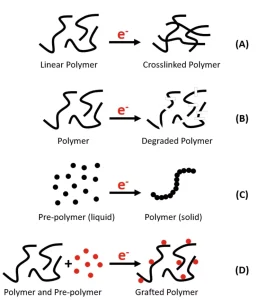
When EB first was commercialized in the 1950s and ’60s, one of the original applications was cross-linking plastic film.3 Cross-linking is the chemical bonding of linear or branched polymer chains into a linked network (Figure 1A). Electron beam cross-links plastics by breaking bonds within the polymer, which then can reform with neighboring chains to produce a net-like structure. No additional chemistry is needed for EB cross-linking – a benefit for both efficient processing and regulated applications, such as medical and direct food contact. By bonding the polymer chains together instead of relying on simple mechanical entanglement, properties such as heat resistance, solvent resistance, tensile strength and shrink are improved.1-3 Often, cross-linking allows for the downgauging of film without sacrificing performance. Pressure-sensitive adhesives can be cross-linked to control the level of tack. Applications of EB cross-linking include shrink films, vacuum skin packaging, cable insulation, tires and tapes.
Not all polymer materials will cross-link under the electron beam; some materials undergo a degradation process called chain scission.3 As with cross-linking, the beam breaks bonds within the polymer, but these bonds remain broken during chain scission (Figure 1B). In reality, cross-linking and scission always occur in concert, and which of the two reactions dominates is dependent on the polymer chemistry. EB sterilization is an example of chain scission: The DNA (a natural polymer) of microorganisms scissions with electron beam exposure, and the microorganisms are destroyed. Electron beam can be used to produce aseptic packaging and to sterilize food products, such as spices, without the use of added chemicals.4 Another example of EB chain scission is the recycling of PTFE, commonly known as Teflon®.3,5 EB is used to degrade PTFE – which can be a difficult material to work with – into a powder form that can be used in lubricants, inks and coatings.
Both cross-linking and chain scission are reactions that start with a polymer. Electron beam also can be used to initiate the polymerization of prepolymers, such as monomers and oligomers, to create polymers (Figure 1C).1-3,6 As the polymer chains are formed during the reaction, generally cross-linking also occurs, resulting in robust coatings, inks and adhesives with low migration and low to no odor. As with other EB reactions, polymerization is a virtually instant reaction, taking place in milliseconds, without initiator or solvent. Because electrons are not governed by optical clarity, it is the density of the material that affects penetration. EB can cure through opaque inks or metallized laminates. Electron beam inks, coatings and adhesives have been formulated for use on a variety of different substrates – including paper, paperboard, film, wood and metal – and with a variety of different print methods, both analog and digital. These attributes have facilitated the adoption of EB polymerization in exterior and interior architectural products, décor paper, paperboard and flexible food packaging, and silicone release materials, among other applications.
When polymer and prepolymer are exposed to the beam together, the result is grafting (Figure 1D).1 The accelerated electrons break bonds within the polymer, creating reaction sites for the prepolymer to bond and polymerize. Grafting has been used extensively for surface modification. For example, a hydrophilic prepolymer can be grafted to a hydrophobic membrane or filter to make a hydrophilic surface without altering the bulk properties of the original membrane.
In addition to the more established applications of electron beam technology, new uses are ever on the horizon. Whether it’s expanding the scope of an existing application – for instance, the inclusion of compostable and recyclable substrates in flexible packaging7 – or developing entirely new applications – e.g., batteries8, gas-to-liquid conversion9 and modification of inorganic materials10 – researchers and companies alike are realizing the vast potential of electron beam technology. This potential further is bolstered by EB’s ability to operate with relatively little energy consumption,11 solvent free and, in some applications, with no added chemicals. This ability makes electron beam inherently a green technology – an important attribute of any emerging application as societies strive for greater environmental consciousness.
The core principle of electron beam technology is accelerated electrons break chemical bonds. What happens afterward (bonds remain broken, reform and/or initiate a reaction) is chemistry. How this principle is used to create new EB applications is up to your ingenuity!
References
- Clough, R.L., 2001. High-energy radiation and polymers: a review of commercial processes and emerging applications. Nucl. Instrum. Meth. B. 185, 8-33.
- Mehnert, R., 1995. Electron beams in research and technology. Nucl. Instrum. Meth. B. 105, 348-358.
- Makuuchi, K., Cheng, S., 2012. Radiation processing of polymer materials and its industrial applications. Wiley.
- Gryczka, U., Kameya, H., Kimura, K., Todoriki, S., Migdal, W., Bulka, S., 2020. Efficacy of low energy electron beam on microbial decontamination of spices. Radiat. Phys. Chem. 170, 108662.
- Burillo, G., Clough, R. L., Czvikovsky, T., Guven, O., Le Moel, A., Liu, W., Signh, A., Yang, J., Zaharescu, T., 2002. Polymer recycling: potential application of radiation technology. Radiat. Phys. Chem. 64, 41-51.
- Fouassier, J.P., Rabek, J.F. (Eds.), 1993. Radiation curing in polymer science and technology. Vol. 3, 301-339.
- Schissel, S.M., 2020. Attainable sustainable: using electron beam technology in compostable flexible packaging. UV+EB Tech. (2) 14-20.
- Du, Z., Janke, C.J., Li, J., Wood, D.L, 2019. High-speed electron beam curing of thick electrode for high energy density Li-ion batteries. Green Enrgy. Envir. 4, 375-381.
- Ponomarev, A.V., 2009. Electron beam radiolysis of gaseous alkanes under circulation conditions: gas-to-liquid transformation. Radiat. Phys. Chem. 78, 48-56.
- Kim, B., Park, J.H., Lee, H.S., Kim, M.M., 2016. Fabrication and characterization of silver nanoparticles by using a low energy electron accelerator with a flow reactor. J. Korean Phys. Soc. 69, 1163-1167.
- Golden, R., 2012. What’s the score? A method for quantitative estimation of energy use and emission reductions for UV/EB curing. RadTech Report. (3), 44-48.
Sage Schissel, Ph.D.
Applications Specialist
PCT Ebeam and Integration LLC
sage.schissel@pctebi.com