By Jennifer Heathcote, business development manager, GEW, Inc.
Optically transparent UV-cured hardcoats, topcoats, overcoats and clearcoats are utilized in numerous plastics decorating processes. As the descriptors suggest, these coatings tend to be clear but also can be tinted or formulated with other visual additives, are applied to the outermost surface of manufactured parts, and serve to provide a high level of physical protection and/or aesthetic enhancement to the primary goods being manufactured. Examples of plastic decorating processes that incorporate UV-cured hardcoats include physical vapor deposition (PVD), in-mold decorating (IMD), in-mold labeling (IML) and finishing.
In PVD processes, metal is vaporized and condensed onto plastic surfaces in very thin layers to produce visually flawless metallized effects. The metal is then protected with a UV-cured clearcoat. For injection and blow molding applications, preprinted thermoformed sheets or flexible film appliqués are inserted into the tooling of in-mold machines that enable preprinted decorative graphics to be directly molded into the surface of plastic components. One side of the printed material often includes a previously cured UV topcoat or is UV cured after thermoforming and before molding.
In finishing processes involving 3D parts, manufacturers spray, dip, flow or vacuum coat protective UV-curable coatings directly onto formed items, which are immediately cured inline. UV topcoats also are instrumental in upstream processes, where they are applied to the surfaces of rolls of raw polymer film, utilizing various web coating application technologies. These UV-cured rolls or converted sheets are incorporated into downstream print and molding processes and/or applied to the surfaces of other manufactured items.
Product designers find UV-cured coatings appealing for several reasons. First, UV coatings enable lightweight plastic parts to be produced with hard and durable outer finishes that can survive handling, filling, assembling, shipping, long-term use and exposure to harsh conditions. It also means that inexpensive plastic materials can be used to create visually appealing items perceived to be of much greater value.
This is possible because printing and decorating processes have been perfected to disguise plastic surfaces so that they appear metallic; exhibit a wood grain or other natural material composition; or display colorful printed graphics, logos, text and symbolic operator interface guidance.
With UV-cured coatings, it is even possible to incorporate added functionality such as the ability for surfaces to be easily cleaned; provide antimicrobial capabilities; resist scratching, abrasion, marring, water and chemicals; enhance reflection or reduce glare; and improve weatherability.
A wide range of plastic goods manufactured for automotive, household and industrial uses require UV-curable hardcoats for the aforementioned reasons. Examples include gas station pump panels; membrane switches; touchscreens; electronic signature pads; natural and backlit advertisement, solar, and architectural panels; automotive interior trim and external assemblies; rigid cosmetic packaging; embellishments and hardware fixtures; medical devices, home interior and lawn and garden equipment panels and assemblies; mobile phones and accessories; lighting louvers; polarized sun and safety glasses; LCD displays for televisions, smartphones, laptops and tablets; and others.
UV-curable polymer coatings are typically applied in a liquid-like state and immediately transformed into a cross-linked solid upon exposure to ultraviolet light. UV curing is a photopolymerization process in which ultraviolet energy drives chemical cross-linking reactions in specially formulated materials. Exposure to UV energy creates incredibly strong molecular bonds within polymer resins in less than a second. These bonds are instrumental in generating the surface properties characteristic of hardcoats. From a processing perspective, UV curing provides the necessary energy to cross-link coatings without transferring excessive thermal heat, which can melt or warp the underlying plastic components. UV-cured coatings also have the advantage of being able to adhere to an extensive range of materials.
Historically, medium-pressure mercury vapor lamps, including both electrode arc and microwave, have been used for curing UV hardcoats. Users of hardcoats have been closely watching the progress UV LED curing has made in structural bonding adhesives, fiber optic coatings, printing inks, pressure-sensitive adhesives and graphics coatings. As a result, plastics manufacturers are anxiously anticipating the availability of UV LED-curable surface coatings for their own processes and are increasingly asking material and equipment suppliers for updates on UV LED hardcoat options.
While UV LED curing technology is not yet ready for most hardcoat applications due to 1) lack of UVC LEDs, 2) oxygen inhibition at the surface, and 3) insufficient irradiance at greater working distances, development activity is increasing in this space and will drive viability and adoption in the future.
UV spectral output
Ultraviolet wavelengths between 200 and 450 nm are fundamental to UV curing and commonly segmented as:
- UVC (200 to 280 nm)
- UVB (280 to 315 nm)
- UVA (315 to 400 nm)
- UVV (400 to 450 nm)
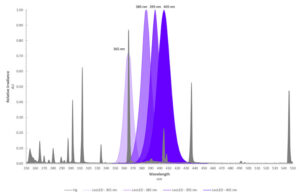
Of these four segments, UVC produces the shortest and greatest energy wavelengths while UVV produces the longest and lowest energy wavelengths. UVC wavelengths tend to be absorbed at the surface of coatings while UVA and UVV wavelengths penetrate much deeper. Medium-pressure mercury vapor lamps are broad-spectrum and emit ultraviolet energy across all four UV bands as well as visible and infrared bands. By comparison, today’s UV LED curing lamps only practically emit longer UVA and UVV wavelengths between 365 and 405 nm, with recent development in UVC occurring between 275 and 285 nm. A comparison of a mercury vapor lamp to UV LEDs is illustrated in Figure 1.
While a given photoinitiator or PI package will absorb ultraviolet energy across a wide range of wavelengths, photoinitiators commonly used in highly functional industrial coatings were historically designed to absorb UV most efficiently between 220 and 260 nm while also reacting somewhat to longer UVC and UVB wavelengths. These particular PIs tend to not absorb any UVA output. As a result, the primary challenge delaying UV LED curing of hardcoats is a mismatch between long UVA wavelengths emitted by commercial UV LEDs and the chemistry’s need for short UVC wavelengths.
In non-hardcoat markets presently using UV LEDs to cure inks, coatings and adhesives, formulators are limited to specific photoinitiators that react to longer UVA wavelengths. Since longer wavelength absorbing PIs tend to yellow when exposed to UV energy, formulators must mask the yellowing with pigments or blend various photoinitiators with other chemistry in ways that minimize yellowing.
While this was not initially straightforward, ongoing development has successfully led to clear overprint varnishes (OPVs) for graphics coatings that cure with LED at competitive line speeds, are acceptably nonyellowing and provide enough protection for many printed labels and some packaging. Unfortunately, the inability to use preferable photoinitiators that react to UVC means that many of the more durable properties delivered by hardcoats are simply not possible with longer wavelength LEDs today.
Surface cure and oxygen inhibition
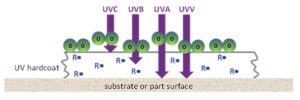
When photoinitiators absorb ultraviolet wavelengths, they create free radicals (R•) as illustrated in Figure 2. Free radicals drive cross-linking by bonding with resin molecules to create long, continuous polymer chains.
Oxygen inhibition occurs when oxygen (O2) molecules in the air interfere with the UV cross-linking process. This interference is generally limited to the surface of the coating, where it weakens free radicals and reduces their overall concentration. Formulations sensitive to oxygen inhibition tend to have incomplete polymerization near the outermost surface. This often results in a sticky, tacky or greasy feeling.
For many mercury vapor curing applications, the presence of UVC wavelengths is enough to counter oxygen inhibition. Since UVC wavelengths are higher in energy and easily absorbed by free radical generating photoinitiators at the surface, they often can produce enough free radicals to offset those directly lost to oxygen (quenched) or weakened by oxygen (scavenged). In cases where UVC wavelengths are completely absent from curing processes or have insufficient power, the environment, formulation and/or lamp head configuration can be modified to improve surface cure.
For example, flooding the surface of the chemistry with a nonreactive gas, such as nitrogen, removes oxygen from the immediate environment and prevents it from interfering with the reaction. Alternatively, increasing the viscosity or thickness of a coating reduces oxygen diffusion and increases free radical generation within the coating. This can result in the outermost top surface being cured from the bottom up. In other cases, the photoinitiator and other reactive chemistry concentrations as well as the irradiance and energy density of the UV system each can be increased in an effort to drive the creation of more free radicals.
For additional information on how oxygen interferes with free-radical reactions, refer to Mitigation of Oxygen Inhibition in UV LED, UVA, and Low Intensity UV Cure by Jo Ann Arceneaux, Ph.D., Allnex USA, Inc.
In general, it is possible to drive irradiance to greater levels with UV LEDs than mercury lamps, as illustrated by the chart in Figure 1; however, once UV output surpasses the minimum threshold irradiance needed to initiate the reaction and offset oxygen inhibition, energy density (J/cm2), which is the total delivered energy over time, has a greater impact on cure.
Irradiance at greater working distances
For three-dimensional parts with drastic or deep part profiles, another challenge for surface hardcoats is the magnitude of the irradiance. UV curing requires direct line of sight between the lamp head and all facets of the cure surface. For webs and flat part profiles, this is not an issue. As part shapes become more complicated, however, lamp heads must be positioned further from part surfaces to provide suitable clearance. Multiple lamp heads positioned at various angles also are sometimes necessary to provide full UV coverage to 3D parts.
Mercury vapor lamps used in part finishing and on web coating applications that experience web flutter and bounce in front of curing lamps often have a 50 mm (2-inch) focal length. This concentrates ultraviolet energy in front of the lamp head by the specified focal distance.
By comparison, UV LED curing systems are a flat matrix of diodes where the greatest irradiance occurs near the lamp emitting window, and for conventional systems utilized in web applications where web tension and curing on rollers eliminates flutter, GEW electrode arc lamp systems utilize a 3 mm (0.12-inch) focal length.
UV LED output is emitted from flat emitting windows at a typical beam angle of 120°. As rays travel away from LED lamp heads, they diverge. The spreading out of rays reduces UV concentration and ultimately decreases the magnitude of the irradiance arriving at the cure surface. A minimum threshold irradiance required by the formulation is necessary to generate free radicals and drive cross-linking.
While commercial UV LEDs have considerably higher peak irradiance values than mercury vapor, the magnitude rapidly diminishes with increasing distance. There are always exceptions, but most commercial UV LED curing applications are designed to keep the UV LED lamp head as close to the web or part surface as possible for this reason.
In other cases, chemistry is designed to react to a lower irradiance, or reflectors and optics are incorporated to collimate or direct the light across a greater distance.
Status of UVC LEDs
From an electronics fabrication perspective, the most promising LED wavelengths within the UVC band lie between 275 and 285 nm. While this is outside the preferred 220 to 260 nm range for hardcoat chemistry, prototype curing systems are currently emitting up to 4 Watts/cm2.
This is considerably higher than mercury vapor UVC irradiance. That said, this peak irradiance is measured at the lamp head and will fall to much lower levels at distances of 25 to 100 mm (1 to 4 inches).
In addition, short wavelength UVC LEDs do not yet satisfy cost, reliability and life requirements. It is important to note that UVA LEDs suffered through these same challenges 15 years ago, all of which were overcome through persistent innovation and development. Over the next five to 10 years, it is anticipated that UVC LEDs will follow a similar development path to what UVA LEDs experienced over the previous 15 years.
As both UV LED curing systems and UV LED chemistry continue to evolve, they will become increasingly more compatible with surface hardcoats and plastic decorating processes. This means that users of hardcoats will eventually reap the many benefits of UV LED curing technology, including longer source life, improved process control and reduced heat transfer to parts.
Until then, mercury vapor lamps and hybrid Arc/LED solutions are the preferred curing source for UV hardcoats. Current users, however, are strongly encouraged to continue communicating interest in UV LED coating technology to suppliers, as market demand is a critical factor in how management teams allocate R&D resources.
Jennifer Heathcote is a subject matter expert on mercury vapor UV curing systems and UV LED technology as well as the use of UV curing across a broad range of graphic arts, product decorating and industrial applications. She is business development manager at GEW, Inc., and can be reached at jheathcote@gewuv.com.