In the preceding two columns, I reviewed the current state and future trajectory of the industry through the perspectives of the June 2024 Rapid+TCT Conference. Announcements in the fourth quarter of 2024 show the continuing trend of financial and organizational restructuring described in the prior columns, and this trend will continue in 2025.
This column examines the advantages and limitations of UV printing technologies in which a laser or projector is used to cure resin to create parts in a layer-by-layer approach. Other than jetting, these techniques are the primary methods for UV additive manufacturing.
In their recent review of digital light processing (DLP) printing techniques for microfluidic analytical devices, Amini and colleagues plot the relationship (shown in Figure 1) between printing area and minimum feature size for a variety of vat polymerization technologies. 1
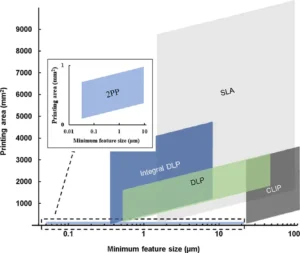
I would suggest that Figure 1 can be used as a tool for comparing 3D printing technologies. For mass-customized medical applications, such as dental appliances and hearing aids, it is more economical to increase the printing area to generate many parts in parallel to minimize the average time required per part. Because stereolithography (SLA) resolution is limited by the UV laser spot size, the SLA print area can be expanded without negatively affecting the minimum feature size. For conventional DLP printers without the collimation optics of integral DLP, increasing the distance between the micromirror array and the vat to accommodate the increasing print area results in an increase in minimum feature size.
The requirements for minimum feature size are dictated by the end-use application. For applications such as dentures, an upper accuracy limit of 120 microns is recommended 2, well within the minimum feature size range of DLP and SLA technology as reported by Amini and colleagues and, therefore, suitable for larger printing areas. For items such as bicycle seats and protective headgear, geometric control of mechanical properties is more critical than minimum feature size. As anticipated from the minimum feature size, the surface quality for vat printing of lenses still requires post-process polishing to achieve optical quality. 3 An alternative approach to direct printing of optical components and other parts requiring submicron feature size utilizes two-photon polymerization, shown in the lower left corner of Figure 1. In conventional UV single-photon photopolymerization, the initiation rate is linearly related to the intensity. 4 Two-photon photopolymerization involves a nonlinear process in which the energy of two photons combines to initiate the reaction. This effect is negligible for conventional UV sources and generally is only observed with focused laser beams as it is proportional to the square of the intensity.
In recently published work, Marx and colleagues report the fabrication of 3.5 mm diameter Fresnel lenses using a laser with a wavelength of 800 nm, a pulse energy of 400 microjoules, a pulse length of 100 femtoseconds and a repetition rate of 5 kHz. 5 Marx uses a substituted benzophenone photoinitiator that does not absorb at 800 nm but performs well at the 400 nm two-photon excitation wavelength. When focused to 2.8 mm, Marx produced lenses with a layer thickness of 100 nm and surface roughness of 15 nm. There also are additional two-photon applications for micron and submicron scale structures in microneedle drug-delivery devices, hydrogel cell scaffolding and biodegradable microbots. 6
I would suggest that the axis corresponding to minimum feature size depicted in Figure 1 also can be interpreted as the scale of mass customization. Dentures and hearing aids, which have customized designs for specific individuals, are on the high end of feature dimensions and low end of cost per part. On the low end of feature dimensions and higher cost per part, objects are identical but designed to accomplish a specific objective, such as controlled drug release for many patients.
Additional additive techniques, such as multimaterial and volumetric 3D printing, as well as applications in the semiconductor industry will be discussed in future
columns.
References
- Amini, A.; Guijt, R.; Themelis, T.; De Vos, J.; Eeltink, S. Recent developments in digital light processing 3D-printing techniques for microfluidic analytical devices Journal of Chromatography A, 2023, 1692, 463842-463855
- Nemeth, A.; Vitai, V.; Czumbel, M.; Szabo, B.; Varga, G.; Keremi, B.; Hegyi, P.; Herman, P.; Borbely, J. Clear guidance to select the most accurate technologies for 3D printing dental models – A network meta-analysis Journal of Dentistry, 2023, 134, 104532-104544
- https://formlabs.com/blog/creating-camera-lenses-with-stereolithography/ (accessed January 7, 2025)
- Lee, J.; Prud’homme, R.; Aksay, I. Cure depth in photopolymerization: Experiments and Theory Mater. Res. 2001, 16(12), 3536-3544
- Marx, J.; Behlau, F.; Haske, D.; Esen, C.; Ostendorf, A. Applied Optics, 2024, 63(24), 6495-6503
- O’Halloran, S.; Pandit, A.; Heise, A.; Kellett, A. Two-Photon Polymerization: Fundamentals, Materials and Chemical Modification Strategies Advanced Science, 2023, 10, 2204072-2204089
Paul Share, Ph.D.
Principal Consultant
Advanced Materials
Design LLC