By Scott R. Sabreen, The Sabreen Group, Inc.
Abstract
The demand for UV-cure inkjet printing and coating onto two-dimensional and three-dimensional plastic products – including thin films – is rapidly increasing due to the advantages offered by full-color digital technology. Arguably, the most significant challenge to achieving robust print quality is inadequate adhesion to polymer substrate. Gas-phase plasma technologies solve inkjet adhesion problems associated with low surface energy and heat-sensitive polymers. This article examines plasma science and the interaction mechanisms between a plasma and polymer surface, and also debunks common mythologies.
Introduction
Inkjet printing is far more complex and delicate than analog printing. Inkjet requires the printhead nozzles to fire precisely sized drops with exact accuracy. UV-cure inks are low in viscosity and surface tension, which creates adhesion bonding problems with the target polymer substrate. Ink shrinkage can be a contributing factor. Companies investing in inkjet technology normally decorate multiple substrates, including stretchable elastomers/rubbers, urethanes, acetals, nylons, polyolefins and more. There are chemical and physical differences between plastics, even within the same polymer family, so it becomes challenging to print effectively on all materials.
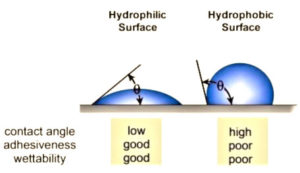
Eco-friendly plasma surface oxidation pretreatments solve adhesion problems and provide a desirable alternative to chemical primers. “Gas-phase” plasma surface modification oxidation, the most common modification method, is proven to be highly effective, economical and environmentally safe. The selection of which method to utilize on any given application can be challenging, in part due to misconceptions and confusing terminology. Commonly known processes are electrical corona, remote-cold gas plasma, flame and UV/ozone (combinations). Significant cost implications are associated with adhesion failures. Through understanding the basic science of contact angles, surface wetting and chemical activation, virtually any adhesion problem can be solved, even when using the most tough-to-bond polymeric and elastomeric materials.
Contact angle, surface energy and wetting
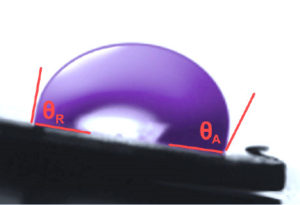
The underlying reasons why many plastics are difficult to bond are because they are hydrophobic non-polar materials, chemically inert and possess poor surface wettability – i.e., low surface energy. While these hydrophobic (water-repelling) performance properties are ideal for part designers who seek such properties, they are the nemesis of manufacturers that need to bond such materials. Robust adhesion usually necessitates hydrophilic surfaces. For optimum adhesion, an ink or coating must thoroughly “wet out” the surface (adherend) to be bonded.
“Wetting out” means the liquid flows and covers a surface to maximize the contact area and the attractive forces between the adhesive and adherend bonding surface. For a liquid to effectively wet out a surface, the surface energy of the adhesive must be as low or lower than the surface energy of the adherend to be bonded. Alternatively, the surface energy of the substrate must be raised. Surface tension, a measurement of surface energy, is expressed in dynes/cm (SI N/m). The higher the surface energy of the solid substrate relative to the surface tension of a liquid (water, printing inks, adhesives/encapsulation, coatings, etc.), the better will be its “wettability” and the smaller will be the contact angle. As a rule, acceptable bonding adhesion is achieved when the surface energy of a substrate is approximately 8 to 10 dynes/cm greater than the surface tension of the liquid. Specific to adhesion of low-viscosity UV inkjet inks onto polymer surfaces, experts find the optimal range of 48 to 56 dynes/cm typically yields better adhesion and print quality. Higher surface energies, greater than 60 dynes/cm, can cause dot separation, particularly on cylindrical products, such as writing instruments and bottles. In other words, achieving 70 dynes/cm (water-wet) is not necessarily advantageous.
Ink shrinkage is an important factor for adhesion and print quality. The volume shrinkage of monomers (acrylates and methacrylates) occurs during polymerization and is due to the replacement of long-distance connections via weak Van der Waals force by strong, short covalent bonds between the carbon atoms of different monomer units. This volume shrinkage causes serious problems, including a large buildup of internal stress, which results in defect formation and dimensional changes, which are responsible for decreased mechanical properties1.
Consider a single liquid fluid droplet on a flat, solid surface at rest (equilibrium). The angle formed by the solid surface and the tangent line to the upper surface at the end-point is called the contact angle. It is the angle (Ø) through the liquid between the tangent line at the contact point and the horizontal line of the solid surface. The bubble/droplet shape is due to the molecular forces by which all liquids, through contraction of the surface, tend to form the contained volume into a shape having the least surface area. The intermolecular forces that contract the surface are termed “surface tension.” Surface tension, a measurement of surface energy, is expressed in dynes/cm (SI N/m).
The higher the surface energy of the solid substrate relative to the surface tension of a liquid (water, printing inks, adhesives/ encapsulation, coatings, etc.), the better will be its “wettability” and the smaller will be the contact angle (Figure 1). As a rule, acceptable bonding adhesion is achieved when the surface energy of a substrate is approximately 8 to 10 dynes/cm greater than the surface tension of the liquid.
For many applications it may be necessary only to examine the static equilibrium contact angle using dyne solutions in accordance to a documented test procedure, such as ASTM D2578. Application kits or “dyne pens/solutions” provide useful information, but they are not precise measurements of surface tension. Surface tension measurements can vary considerably by individual (technique) and the interpretation of the “center” liquid behavior. Dyne pens/solutions are known to be directional indicators of significant differences in the surface tension and capable of identifying “good” and “bad” bondable surfaces at economical pricing. Bottled dyne solutions are preferable to pens because of contamination issues with multiple uses.
Dynamic contact angles
Testing the fluid behavior of only the static contact angle can lead to misinterpretation of the liquid/solid interface results and the resolution of adhesion problems. This is because industrial inkjet production operations are more realistically dynamic conditions, not static. Thus, the dynamic contact angle (DCA) is important to understand (Figure 2).
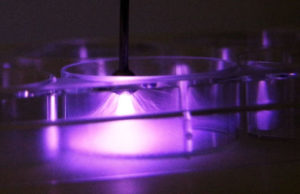
Contact angles generally are affected both by changes in surface chemistry and changes in surface topography. The advancing contact angle is most sensitive to the low-energy (unmodified) components of the substrate surface while the receding angle is more sensitive to the high-energy, oxidized groups introduced by surface pretreatments. Thus, the receding angle is the measurement most characteristic of the modified component of the surface following pretreatments, as measured using dyne solutions. Therefore, it is important to measure both the advancing and receding contact angles on all surface-modified materials.
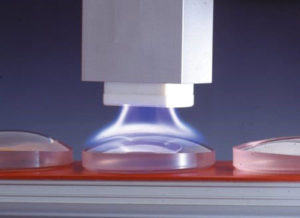
courtesy of 3DT LLC.
When a droplet is attached to a solid surface and the solid surface is tilted, the droplet will lunge forward and slide downward. The angles formed are respectively termed the advancing angle (θa) and the receding angle (θr). ASTM D724 describes methods for measuring dynamic contact angles (DCAs) using advanced equipment (optical tensiometers and goniometers) to analyze advancing and receding contact angles based on drop shape analysis and mass.
Chemical surface activation
There is a strong tendency for manufacturers to focus solely on contact angles or other wettability measurements as the single predictor for adhesion problems and for conducting routine surface testing. Chemical surface functionality is equally important, whereby hydrophobic surfaces are activated into bondable hydrophilic surfaces. Gas-phase, “glow-discharge,” surface oxidation pretreatment processes are used for chemical surface activation. These processes are characterized by their ability to generate “gas plasma,” an extremely reactive gas consisting of free electrons, positive ions and other species. Plasmas often can be described as a fourth state of matter. As energy is supplied, solids melt into liquids, liquids vaporize into gases and gases ionize into “plasmas.”
In the science of physics, the mechanisms in which these plasmas are generated are different, but their effects on surface wettability are similar. The basic chemical and physical reaction that occurs is free electrons, ions, metastables and radicals. UV generated in the plasma can impact a surface with energies sufficient to break the molecular bonds on the surface of most polymeric substrates. This creates very reactive free radicals on the polymer surface which, in turn, can form, cross-link or – in the presence of oxygen – react rapidly to form various chemical functional groups on the substrate surface. Polar functional groups that can form and enhance bondability include carbonyl (C=O), carboxyl (HOOC), hydroperoxide (HOO-) and hydroxyl (HO-) groups. Even small amounts of reactive functional groups incorporated into polymers can be highly beneficial to improving surface chemical functionality and wettability. Mechanical abrasion (including mold tool texture) can be utilized in conjunction with plasma pretreatments.
Commonly known processes are electrical corona discharge (also known as a dielectric barrier discharge), electrical atmospheric plasma, electrical air plasma, flame plasma, low-pressure RF cold gas and ultraviolet irradiation/ ozone (combinations). Each method is application-specific and possesses unique advantages and potential limitations. Examination of the polymer (amorphous or semi-crystalline), plasma process, bonding agent and manufacturing process requires careful investigation and testing. One important study conducted on polypropylene found that flame treatment appears to be the most “shallow”; that is, the oxygen incorporated by the treatment is most concentrated near the outer surface of the film. Corona and plasma treatments appear to penetrate somewhat deeper into the polymers. At the other extreme, the UV/ozone treatments reach farther into the bulk of the polymers. Discharge conditions, such as humidity effects, also are important1. It is known that certain pretreatments and how they are applied can deleteriously affect downstream manufacturing operations, e.g., delamination of toothpaste tubes during heat-sealing.
Plasma surface pretreatments
Classical electrical corona discharge (dielectric barrier discharge) is obtained using a generator and electrode(s) connected to a high-voltage source, a counter electrode at potential zero and a dielectric used as a barrier (Image 1). That is, a high-frequency, high-voltage discharge (step-up transformer) creating a potential difference between two points, requiring earth-ground 35+ kV and 20 to 25 kHz. Custom electrode configurations allow for treating many different surface geometries – flat, contoured, recessed, isolated, etc. One application example is a corona discharge treating system for electrical connectors in which a combination of pin and ball electrodes concomitantly treats 3D small-diameter holes and flat exterior surfaces, US Patent US5051586 (1991)2. Ozone is produced in the plasma region as a result of the electrical discharge. Corona discharge has been found to be effective at cleaning the invisible hydrocarbon materials present after solvent or detergent washing.
Myth: Atmospheric plasma is a low-cost replacement technology for corona discharge.
Fact: Corona discharge often is more effective for treating larger surface areas and at greater surface depth. In fact, many atmospheric plasma systems are more expensive.
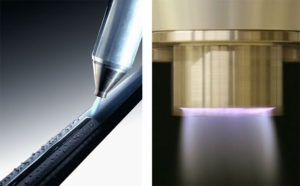
Electrical “air plasma” is a corona discharge spot treatment (also termed blown air plasma/forced air corona/blown arc). This treatment head consists of two hook electrodes in close proximity to each other connected to a high-voltage transformer generating an electric arc of approximately 7 to 12 kV, and lower frequency, 50 to 60 cycles/sec (relative to electrical corona discharge). Then using forced air, a continuous electric arc produces a corona discharge, “plasma” (Image 2). No positive ground is needed. This pretreatment process has virtually no cleaning capabilities. Ozone is produced.
Myth: Corona discharge spot treatment yields longer shelf life than flaming.
Fact: Scholarly studies show flame plasma on polyolefins produces longer post-treatment shelf life due to its relatively shallower depth of treatment.
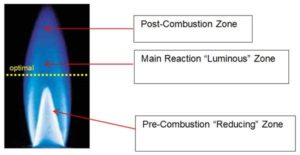
Atmospheric plasma or electrical blown ion plasma (also termed focused corona plasma) utilizes a single narrow-nozzle electrode powered by an electrical generator and step-up transformer along with high-pressurized air (Image 3). Intense focused plasma is generated within the treatment head and streams outward. A recent advancement is variable plasma discharge power control. Adjustable power is an important feature when treating sensitive and/or thin polymeric substrates and printing low-viscosity inks. Adjustable (variable) plasma discharge power can be accomplished in a couple of scholarly reviewed methods, including varying formations of the signal input (i.e., changing frequency) or varying the number of pulse signals applied to parallel electrodes in unit time, termed pulse-density-modulation (PDM). The former method is typically incorporated when output power exceeds 100 watts. The latter method is highly effective for steady-state plasma power control below 100 watts. Atmospheric plasma pretreatment process can clean dirt, debris and some hydrocarbons from the substrate – but not most silicones and slip agents. Research indicates that fine etching of the surface can create new topographies for increased mechanical bonding. Ozone formation is negligible.
Myth: Atmospheric plasma is electrically potential-free.
Fact: Atmospheric plasma is better characterized as “low potential” unless definitively proven to be zero on any specific application. Performance differences exist among equipment manufacturers.
Flame plasma treatment uses the highly reactive species present in the combustion of air and hydrocarbon gas to create the plasma (Image 4). While flame treatment is exothermic, heat does not create the chemical functionality and improved surface wetting. Flaming will clean dirt, debris and some hydrocarbons from the substrate. Flaming will not remove silicones, mold releases and slip agents. Flame treatment can impart higher wetting, oxidation and shelf-life than electrical pretreatments due to its relative shallower depth of treatment from the surface, 5 to 10 nm. Ozone is not produced. When procuring flame treatment burners, compare ribbon versus drilled port and the benefits of zero-balanced regulators.
Myth: Flame treating is unsafe. False criticism arises from competitive equipment manufacturers.
Fact: For decades, flame treating has been used as a safe and effective technique across many industries.
Pyrosil® is a newly evaluated pretreatment optimized for plastics and metals (Figure 3) by Applied Surface Technologies LLC and The Sabreen Group Inc. This technology is different than standard flame treatment. The process is a proprietary flame technology developed by SURA Instruments GmbH 30 years ago and is effective for pretreating glass and ceramics. The process has been independently advanced for plastic applications that require UV inkjet adhesion and decorative/functional coatings. In some instances, the process outperformed traditional electrical discharge and flaming methods. Proper controls of the primer application and flame combustion are critical for adhesion. Some users tend to apply excess primer, which results in less than optimal adhesion and unnecessarily increases costs.
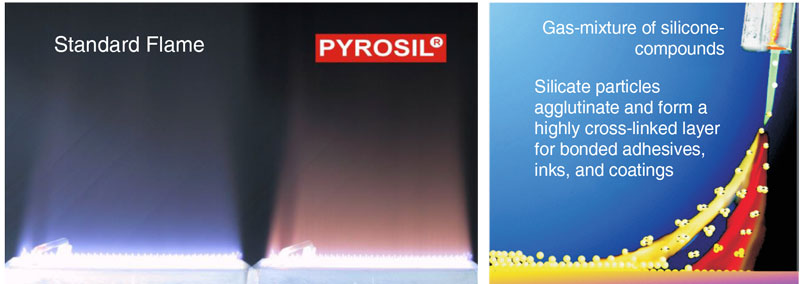
The process is a combustion chemical vapor deposition technology leading to an amorphous silicate layer on the treated substrate. The surface is treated with the oxidizing part of a gas flame, which contains the precursor, an organosilicon compound. The precursor is pyrolysed (thermal decomposition) during the process, and the formed “ash” is deposited as amorphous silicate on the surface, leading to an ultra-thin (20 to 40 nm), strongly adhering coating. In other words, a chemically highly reactive glass-like layer is formed. By evaporating a proprietary chemistry, which is mixed with propane and then burned, silicon dioxide (SiOx) is deposited onto substrates. SiOx creates high surface tension for improved hydrophilicity (wettability). Easily scalable production systems can be custom-built.
Myth: Pyrosil® process is the same as traditional flame treatment.
Fact: The technology is different than flame treatment, i.e., a deposition process mixture of gas and silicone compounds.
Cold gas plasma, also termed “low pressure cold gas plasma,” is conducted in an enclosed evacuated chamber, in comparison to atmospheric (air) surface pretreatment methods (Figures 4a and 4b). Industrial-grade 100% oxygen gas (O2) commonly is used, as well as other gases and combinations of gases. Gas is released into the chamber under a partial vacuum and subjected to a radio frequency (RF) electric field. It is the response of the highly reactive species generated with the polymers placed in the plasma field on inner conductive electrode aluminum shelves or cage, breaking molecular bonds, that result in cleaning and chemical/physical modifications (including an increase in surface roughness, which improves mechanical bonding). A significant benefit of cold gas plasma processes is the removal of hydrocarbons, thereby eliminating solvent cleaning. Atmospheric pretreatments do not remove/clean all poly-aromatic hydrocarbons, so solvent cleaning (prior to pretreatment) may be necessary.
Myth: Cold gas plasma batch processing is too slow when compared to inline treatment methods.
Fact: Large volumes of parts often can be pretreated (batch) and fed into automated assembly operations, requiring no additional processing. Cold gas plasma-treated parts tend to demonstrate the highest quality treatment and longest shelf life. Criticism arises from competitive equipment manufacturers.
Factors influencing adhesion and aging (shelf life)
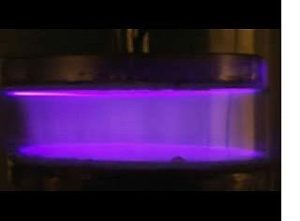
The degree or quality of pretreatment for robust adhesion strength is affected by the cleanliness of the plastic surfaces. The surface must be clean to achieve optimal pretreatment and subsequent adhesion. Contamination sources on product surfaces that inhibit treatment include dirt, dust, grease and oil. Low molecular weight materials, such as silicones, mold release and anti-slip agents, are particularly deleterious for bonding. Material purity also is an important factor. Certain soluble or nonsoluble compound agents used in pigment and dye colorants can adversely affect adhesion.
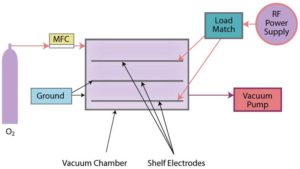
The shelf life (treatment aging degradation) of treated plastics depends on the type of resin, formulation and ambient environment of the storage area. Shelf life of treated products is limited by the presence of low molecular weight oxidized materials (LMWOM) such as antioxidants, plasticizers, slip and antistatic agents, colorants and pigments, and stabilizers, etc. Exposure of treated surfaces to elevated temperatures increases molecular chain mobility. The higher the chain mobility, the faster the aging of the pretreatment. Plasma-treated surfaces age at different rates and to varying extents relative to factors within the surrounding environment. Aging characteristics and storage shelf life are essential to manufacturing process operations. Activated surfaces may have a shelf life of hours, days, months or longer. It is recommended to bond, coat, print, paint or decorate products as soon as possible after pretreatment.
Selecting a plasma surface pretreatment
Recognize that each surface pretreatment method is application-specific and may possess unique advantages and potential limitations. Consider the following factors:
- Polymers react differently to oxidation processes. The type of polymer and its end use performance requirements are critical in determining the selection of pretreatment method.
- Is the substrate (product to be treated) conductive? For example, unassembled plastic electronic connector bodies – without metal contact pins – can be treated electrically; whereas assembled connectors may experience electrical arcing problems.
- Consider part geometry. Flat surfaces are more easily treated compared to deep recesses, extreme tapers and other shape irregularities. Wetting tests are difficult to conduct in small areas and on heavily textured surfaces.
- Is material handling automated? Conductive belts and chains may cause electrical arcing with classical electrical corona discharge and spot treaters. Alternatively, consider using flame, cold gas plasma or low potential atmospheric plasma.
- Avoid overtreatment. Excessive plasma-oxidation of surfaces may deleteriously affect downstream assembly processes, resulting in poor heat sealing/welding.
- All pretreatment equipment is not created equal. Examine the quality of constructed systems in action. For electrical treatment processes, observe the uniformity of the plasma discharge; for flame treatment, consider the differences between ribbon versus drilled port burners and combustion system components; for cold gas plasma, examine the quality of the chamber construction, electrode shelves and, particularly, the manufacturer of the vacuum pump.
Summary
A significant challenge to achieving robust inkjet printing quality is poor adhesion to polymer substrate. UV-cure inks are low in viscosity and surface tension, which creates bonding problems with the target polymer substrate. For optimum adhesion, an ink or coating must thoroughly “wet out” the surface (adherend) to be bonded. It is important to examine both static and dynamic contact angles. Gas-phase plasma technologies solve inkjet adhesion problems associated with low surface energy and heat-sensitive polymers. The selection of which method to utilize on any given application can be challenging. Through understanding the basic science of contact angles, surface wetting and chemical activation, virtually any inkjet adhesion problem can be solved, even when printing onto the most tough-to-bond polymeric and elastomeric materials.
References and Acknowledgements
- Shrinkage of UV Oligomers and Monomers, Andreas Moeck, RadLab AG, 8956 Killwangen, Switzerland
- Mark Strobel, Mary Jane Walzak, Josephine M. Hill, Amy Lin, Elizabeth Karbashewski and Christopher S. Lyons (2012) A comparison of gas-phase methods of modifying polymer surfaces, Journal of Adhesion Science and Technology, 9:3, 365-383, DOI: 10.1163/156856195X00554
- Scott R. Sabreen, The Sabreen Group, Inc., “Surface Wetting Pretreatment Methods,” Plastics Decorating, 2002
Scott R. Sabreen is founder and president of The Sabreen Group, Inc., an engineering company specializing in secondary plastics manufacturing processes – product security, laser marking, surface pretreatments, bonding, decorating and finishing. Sabreen also is responsible for the advancement of Pyrosil® as a method of plastic pretreatment. He can be contacted at 972.820.6777 or by visiting www.Sabreen.com.