By Nathan Noyes and Wouter Vogel, Ph.D., Croda
Within polymer-based additive manufacturing, photocurable resin-based processes have gained acceptance due to advantages in printing time, surface finish and interlayer adhesion relative to filament or powder fusion techniques. Still, serial production of functional parts via these 3D printing processes is isolated due to material limitations. Through modification of benchmark photocurable resins with bio-based (meth)acrylate derivatives based on dimer fatty acid technology, improvements in dimensional stability, water absorption and toughness have been demonstrated. This enables greater functionality and durability of printed parts.
Introduction
3D printing, or additive manufacturing, is experiencing continuing growth as an alternative to conventional injection molding and subtractive manufacturing processes, in part because the technique allows finely detailed articles of complex geometry to be created – and redesigned, if necessary – without the costly and time-consuming manufacture of molds and with minimal wasted material. For these reasons, 3D printing has been adopted readily for rapid prototyping (e.g. design for automotive parts and consumer goods) and when customization is of high value (e.g. dental molds, orthopedic aids, personalized footwear or athletic gear, and reproduction of nonstock replacement parts). Continual advancements in printing processes and machinery push applications steadily from custom, short-run production closer to serial manufacturing. Of the major printing techniques – including metal sintering, filament deposition, powder sintering and photopolymer processing – the latter accounts for the largest share of material sales to date and is also one of the more useful techniques for creating fully functional articles1. The ability of the energy source in photopolymerization, vat polymerization, process stereolithography (SLA), digital light processing (DLP) or multi-jet systems (MJF/MJM) to penetrate through multiple layers of polymer leads to a matrix with greater cross-linking across the build direction than can be achieved with melt fusion alone.
Still, 3D photopolymerization comes with its own set of material-related limitations. Articles printed via SLA/DLP typically require post-processing to remove excess or incompletely reacted resin as well as support structures. The acrylate resins available are typically rigid and brittle, and control over the cross-link density and flexibility is desired. In addition, the advancement of plastic manufacturing of this kind is affected by sustainability considerations related to circularity or renewability of the raw materials and final polymers.
Use of bio-based resins has gained considerable interest in recent years, with acrylated soybean oil as an example. Also, bio-based (meth)acrylate functional derivatives of specialty dimerized fatty acids have arisen as a platform technology capable of improving on these limiting performance factors. The underlying building block is a branched C36 diacid which, when incorporated into a resin, introduces flexibility, improves melt flow and wetting for interlayer adhesion, and increases durability through reduction in water/chemical absorption. A series of dimer diacrylates also shows exceptional conversion rates and dimensional stability in 3D printing and post-curing.
Cure Conversion and Dimensional Stability
A variety of acrylate and methacrylate monomers and oligomers can be utilized in photopolymer printing. It is generally observed that acrylate components are quick to react2, but methacrylate components result in polymers with comparatively improved mechanical and tensile proprieties3. For this reason, dimethacrylates are typical components of a resin formula. High molecular weight dimethacrylates or lesser functional monomers have been shown to reduce shrinkage during photopolymerization – a factor critical in additive manufacturing – due to a lower relative concentration of reactive sites. However, this may contribute to incomplete conversion. Unreacted monomer is both an occupational risk to users and detrimental to the aesthetics, durability and biocompatibility of printed articles4. In addition, traditional high molecular weight dimethacrylates often are of high viscosity, which impedes printability, as conventional printing with UV resins has a viscosity range of 1 to 10 Pa•s.

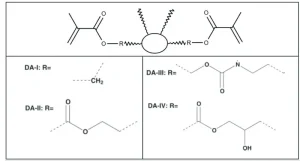
Irregularly structured C36 dimer acid-derived diacrylates (Figure 1), with their aliphatic hydrocarbon core and low crystallinity, maintain a low monomer viscosity, high conversion rate and minimal shrinkage. Such dimer diacrylates can and have been prepared by several synthetic routes, including the series described in Figure 2, prepared by Trujillo-Lemon, et al, from hydrogenated dimer acid5. The dimer acid may be additionally functionalized or polymerized prior to acrylation to enable formulation versatility.
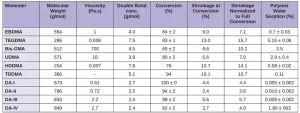
In the homopolymerization of the traditional monomers (carried out with visible light activation using a camphorquinone and ethyl 4-N,N-dimethylaminobenzoate photoinitiator system), higher conversion rates correlated roughly with greater shrinkage. Dimer-acid based monomers generally have both higher conversion rates and lower actual and normalized shrinkage (Table 1). Despite all the tested dimer diacrylates polymerizing to a greater degree than the other monomers, shrinkage remained low, due in part to lower initial double bond concentrations.
Mechanical Improvements
For analysis of dimer diacrylates in a formulated resin, a high bio-based content resin derived from epoxidized soybean oil was selected. Specifically, the chosen resin (referred to here as Bio-Resin) is a 76% bio-based mixture of acrylates and methacrylates determined to meet viscosity requirements for 3D printing, which possesses a higher deformation energy than some industrial-standard SLA resins6. One such standard commercially available clear prototyping resin (StdPR48) is included for comparison. Fractions of two dimer acrylates were compounded to the BioResin: an acrylate of high purity, fully amorphous dimer diol (DDA) and an acrylate of a bio-based amorphous polyester polyol dimer-based diacrylate (pre-polymer, PPDA). In this particular system, the petrochemical fraction is coming from the monomer isobornyl methacrylate (IBOMA), which has been used to modify viscosity for printing, as well as cross-link density.
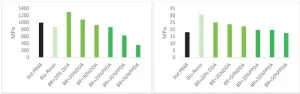
Printed tensile bars were tested in the z-direction for mechanical properties, as shown in Figure 3. A minority addition of dimer diol acrylate improved the modulus of the bio-resin to greater than that of the standard reference acrylic resin, with reductions in modulus observed with the amorphous variant of higher molecular weight. Strain at
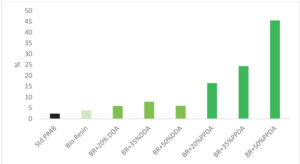
break also decreased with the addition of the bio-based building block. Elongation at break increased in all cases, with the most dramatic increases observed with larger fractions of polyester polyol acrylate.
The combination of improved modulus and flexibility indicates improved toughness of the printed part, which is to be further validated in pending impact testing.
Extended Duration of Functional Part Usable Lifetime
The aliphatic nature of the bio-based dimethacrylates is also known to reduce water absorption and improve resistance to degradation from exposure to chemicals and UV energy or weathering.
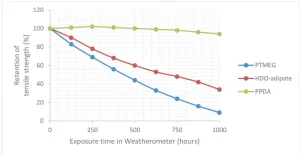
For example, a photocured thermopolastic urethane acrylate was prepared with several different polyols: polytetramethylene ether glycol (PTMEG), hexanediol adipate (HDO-adipate) and paraphenylenediamine (PPDA). Tensile bars were molded and cured before being submitted to a climate chamber for accelerated exposure testing, as shown in Figure 5. Only the urethane acrylate that included the dimer-based polyester polyol retained tensile strength over 1,000 hours, resisting thermo-oxidation, hydrolysis and UV.
Conclusion
Dimer-based acrylate resin modifiers offer a platform to increase bio-based content and improve initial mechanical and aesthetic properties as well as durability of photopolymerized 3D-printed materials. Several monomers have been demonstrated and studied, prepared by a variety of synthetic approaches. Ongoing studies will allow optimization of the renewable photoreactive building block for a given application or resin system.
Wouter Vogel is a research team leader for Croda Smart Materials and New Technologies. He is responsible for synthesis of new polymeric systems, exploring chemical conversions and tuning material properties by molecular design. While completing his doctorate, he spent half a year at the University of North Carolina at Chapel Hill to broaden the scope of his thesis on all-aromatic hyperbranched polymers.
Nathan Noyes spent his first five years with Croda in chemical research and technology, with a focus on applications of sustainable polymer building blocks and performance additives for both thermoset and thermoplastic systems, including for use in additive manufacturing. He is responsible for business development and strategic marketing for the North American region. For more information, visit www.croda.com/en-gbo.
References
- Wohlers Associates. (2016). 3D Printing and Additive Manufacturing State of the Industry: Annual Worldwide Progress Report.
- Ligon, S.C., Liska, R., Stampfl, J., Gurr, M., & Mülhaupt, R. (2017). Polymers for 3D-printing and Customized Additive Manufacturing. Chemical Reviews Vol. 117, 10212-10290.
- deLombard-Watts, M., & Weissman, P.T. (2004). Comparison of performance of acrylate and methacrylate aliphatic urethanes. RadTech.
- Lefeuvre, M.; Amjaad, W.; Goldberg, M.; Stanislawski, L. Biomaterials 2005, 26, 5130–5137.
- Trujillo-Lemon, M., Ge, J., Lu, H., Tanaka, J., & Stansbury, J. (2006). Dimethacrylate Derivatives of Dimer Acid. Journal of Polymer Science, 44. https://doi.org/10.1002/pola.21493
- Guit, J. (2019). Developing Biobased Photopolymer Resins for Stereolithography 3D Printing: Synthesis of Biobased Methacrylate Oligomers and 3D Printing of Formulated Photopolymer Resins. NHL Stenden University of Applied Sciences, GreenPAC, Emmen.