In Parts 1 and 2 of this series on intermolecular attractive forces in polymers, discussions of London Dispersion Forces (LDFs)1 and dipole-dipole attractions2, respectively, were presented. In Part 3, we will discuss an unusually strong type of dipole-dipole attraction – one so strong that it generally is classified as a separate type of intermolecular attractive force: hydrogen-bonding!
For hydrogen-bonds (H-bonds) to exist, a hydrogen atom must be bonded directly to one of the three most electronegative elements on the Periodic Table. With reference to the Allred-Rochow Scale of Electronegativity3, the most electronegative element is fluorine (F), followed in decreasing order by oxygen (O) and nitrogen (N). No other element has a sufficiently high electronegativity difference with hydrogen to form H-bonds among molecules. So, C-H or S-H or Si-H covalent bonds, for example, do not produce H-bonding.
Electronegativity is a relative measure of the force of attraction for electrons being shared in a covalent bond. An electronegativity difference between two atoms bonded to each other causes the bond to be polar, with the negative end of the dipole being on the atom with the higher electronegativity. One simplistically can think of the shared electrons as sitting closer to (or spending more time around) the more electronegative atom, giving that atom a partial negative charge.
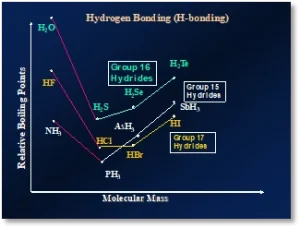
In general, the boiling points increase as the central atom gets bigger. This is due to an increase in London Dispersion Forces among the molecules. So, for Group 17, going from H-Cl to H-Br to H-I, the boiling points increase in spite of the fact that the polarity of the bonds decreases (Figure 1). The same is true for the hydrides of the corresponding elements of Groups 16 and 15, respectively. But note in each case that the first member of the group provides a large exception to this trend. HF, H2O and NH3 all deviate significantly in boiling points compared with their respective congeners. This is strong experimental evidence for H-bonding when hydrogen is bonded to F, O or N. A similar plot (Figure 2) of the relative boiling points of Group 14 hydrides (CH4, SiH4, GeH4 and SnH4) shows no such exception for carbon, the first and smallest member of the group. The relative boiling points of the Nobel gases also are shown in Figure 2 to illustrate the trend.
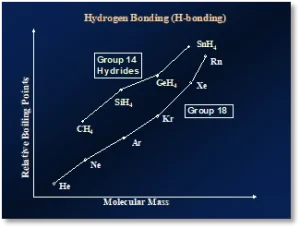
So, why are H-bonds so much stronger than other types of dipole-dipole attractions? The answer involves the fact that hydrogen atoms are so much smaller than any other atom that might be bonded to F, O or N. Hydrogen contributes its one and only electron to the bond. When other atoms contribute their valence electrons to a polar covalent bond, they have one or more electrons left that are not shared with the more electronegative atom. Now imagine, hypothetically, that when an atom contributes one or more of its valence electrons to a shared covalent bond that it gives them up completely to the more electronegative atom! A positive ion would be formed! If the atom giving up its valence electron is hydrogen, it would leave behind a bare proton – a species that would have an enormous positive electric field emanating from it, due to its extremely small size (~10-13 cm). Any other atom would be significantly larger (~10-8cm) in this hypothetical situation and, thus, would not exert such a strong attraction to its negative counterpart on an adjacent molecule. Of course, ions are not actually formed in such cases, so this is merely an analogy. While the hydrogen atom remains attached and does have a share in the bonding electrons, its small size causes its partial positive charge to be larger than that of larger atoms.
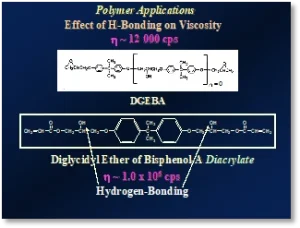
It is important to note that H-F, H-O and H-N bonds are not, in and of themselves, H-bonds! H-bonding occurs when the H-F, H-O or H-N bond then is attracted to the same or similar functional group on an adjacent molecule, e.g., F-H….F-H, O-H….O-H or F-H….O-H. (The H-bonds in these examples are represented by the dashed line [….].)
Relative boiling points, heats of vaporization, melting points, etc., are all thermodynamic properties that can be used to illustrate H-bonding. But viscosity effects also may also be used to demonstrate the power of H-bonding. A classic example is demonstrated by epoxy-based oligomers used in UV/EB polymerization. Figure 3 illustrates this for a diglycidyl ether of bis-Phenol-A and its corresponding diacrylate oligomer4. When the two epoxide groups on the base resin are acrylated with acrylic acid, the diacrylate oligomer is formed. In the process, the viscosity increases from about 12 000 cps to around 1 million cps! This is due to the formation of the secondary O-H groups that form during acrylation. These groups then form H-bonds with adjacent oligomer molecules.
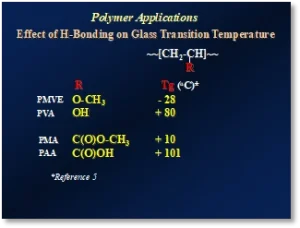
Glass transition temperature (Tg) data also illustrate the power of H-bonding in polymers. Figure 4 provides Tg data for four polymers, two of which exhibit H-bonding and two that do not5. The Tg of poly(vinyl methyl ether) (PVME) is contrasted with that of poly(vinyl alcohol) (PVA), as is the Tg of poly(methyl acrylate) (PMA) with that of poly(acrylic acid) (PAA). In the latter case, the Tg increases by about 90° C and, in the former, it increases by nearly 110° C.
Summary and Conclusions
When evaluating key properties of polymers and distinguishing those properties from those of lower molecular mass materials, intermolecular attractive forces are critical to understanding those properties. London Dispersion Forces (LDFs) are present among atoms, molecules and/or ions in all solids and liquids, whether as pure substances (elements or compounds) or mixtures thereof. Additionally, dipole-dipole attractions exist in species containing polar molecules. Finally, some materials contain molecules that exhibit particularly strong dipole-dipole attractions, known as hydrogen-bonds. These molecules contain functional groups where a hydrogen atom is covalently bonded to fluorine, oxygen or nitrogen, the three most electronegative elements in the Periodic Table. For polymers, these intermolecular attractive forces have significant effects on properties such as cohesive energy density, viscosities in the liquid state, melting temperatures in the solid state (linear and branched polymers), glass transition temperatures (Tgs) and others. Raw material suppliers, formulators and end-users of UV/EB-polymerizable materials all can benefit from a deeper understanding of the intermolecular attractive forces present in polymer samples.
Technical Questions?
What are your technical questions about polymer science, photopolymerization or other topics concerning the chemistry and technology of UV/EB polymerization? Please submit your questions or comments via email directly to Dianna Brodine, Vice President, Editorial for Peterson Media Group at [email protected].
References
- Christmas, B., “Intermolecular Attractive Forces: The Key to Understanding Polymer Properties – Part 1”, UV+EB Technology, Vol. 7, No. 4, Quarter 4, 2021, pp. 16-17.
- Christmas, B., “Intermolecular Attractive Forces: The Key to Understanding Polymer Properties – Part 2”, UV+EB Technology, Vol. 8, No. 1, Quarter 1, 2022, pp. 18-19.
- Huheey, James E., Inorganic Chemistry: Principles of Structure and Reactivity, Harper & Row, pg. 160, 1972.
- Baig, N., Truong, M., Dumas, T., and Christmas, B, “A Simple Process for Preparing Low Viscosity Diacrylate Functional Epoxy Oligomers”, RadTech 2012 UV/EB Conference Proceedings, Chicago, IL.
- http://polymerdatabase.com/polymer%20physics/Polymer%20Tg.html
Byron K. Christmas, Ph.D.
Professor of Chemistry, Emeritus
University of Houston-Downtown
[email protected]