By Robert Rae, managing dir.-sales; Ryan Turner, R&D engineer; and Jennifer Heathcote, business development mgr., GEW
The Earth’s atmosphere is a delicately balanced mixture of nitrogen (N2), oxygen (O2) and argon (Ar) gases with trace amounts of carbon dioxide (CO2) and neon (Ne). Precise concentrations vary slightly depending on elevation from sea level but generally are 78% nitrogen, 20.9% oxygen and 0.93% argon. To exist and function properly, humans require oxygen concentrations between 19.5 and 23.5%. Anything less causes rapid mental, physical and respiratory impairment. Slightly greater concentrations do not necessarily cause health issues but increase the likelihood of environmental hazards such as fires and explosions.
An important property of oxygen is that it reacts with numerous other elements to facilitate a wide range of both beneficial and detrimental biological, chemical and environmental processes. By contrast, nitrogen and argon typically do not react with other substances and are classified as inert. In terms of human life, oxygen is essential. When it comes to free-radical UV curing, however, the presence of oxygen within formulations – and more critically, at the surfaces of formulations – has the potential to adversely affect photopolymerization. This undesirable interference is termed oxygen inhibition.
Oxygen inhibition
Photopolymerization, or UV curing, is a chemical reaction that uses ultraviolet energy to transform specially formulated liquid-like materials that are wet to the touch into solid polymers that are dry to the touch. Photoinitiators (PI) within inks, coatings, adhesives and extrusions absorb UV wavelengths and produce energized free radicals. Free radicals transfer energy by reacting with other free radicals and materials within formulations, such as monomers and oligomers, to create crosslinked polymers. The total reaction occurs within a fraction of a second, producing fully cured materials immediately ready for further processing or shipping.
Oxygen inhibition occurs when oxygen molecules (O2) disrupt the crosslinking process and cause premature termination of polymer chains. When oxygen is present, its molecules behave like a monomer, react with energized free radicals and sabotage the desired propagation of the reaction. This interference mostly occurs at the surface of the coating where an ample supply of oxygen in the atmosphere weakens free radicals and/or reduces overall concentration.
Formulations sensitive to oxygen inhibition struggle to fully polymerize when cured in open air. This can lead to surface cure issues that exhibit characteristics often described as sticky, tacky or greasy. Surface-cure issues sometimes produce lingering odors due to unreacted raw materials; higher degrees of rub-off or smearing, which results in offsetting and tracking on substrates, parts and machine components; and diminished functional properties in the cured polymers.
Various countermeasures
For mercury-vapor curing applications, the presence of UVC wavelengths often is enough to counter oxygen inhibition. Shorter UVC wavelengths (200 to 285 nm) have greater photonic energy compared to longer UVA wavelengths (315 to 400 nm) and easily are absorbed by free-radical-generating photoinitiators at the formulation surface. As a result, UVC wavelengths emitted from industrially rated UV-curing systems typically generate enough free radicals at the surface to offset those directly lost to oxygen (quenched) or weakened by oxygen (scavenged). Consequently, many UV-curing processes using broadband sources are relatively unaffected by oxygen inhibition.
In situations where curing systems emit insufficient or no UVC output, applications where low coatweights yield large surface areas in comparison to laydown thicknesses or cases where a greater crosslink density is desired, it often is necessary to modify the environment, formulation and/or lamphead output to improve cure. The challenge with low-coatweight applications is that a larger portion of the formulation’s total volume is directly exposed to Earth’s atmosphere, making it easier for oxygen to rob the process of energized free radicals. While counterintuitive, thinner laydowns are more challenging to cure than thicker laydowns, and it has everything to do with oxygen inhibition.
There are various ways to counter oxygen inhibition in UV processes. For example, flooding the surface chemistry with a non-reactive gas such as nitrogen forces air and its 20.9% concentration of oxygen away from the immediate environment. With an optimal inert-gas quantity, the level of oxygen is sufficiently reduced to prevent interference within the crosslinking reaction. Alternatively, increasing viscosity or thickness of a formulation reduces oxygen diffusion and increases free-radical generation within the coating. This can result in the outermost “top” surface being cured from “the bottom up.” In other cases, photoinitiators and other reactive chemistry concentrations – as well as the irradiance and energy density of UV-curing systems – each can be increased to drive the creation of more free radicals. The goal here is to outpace oxygen inhibition. This is not without limit, however, as increasingly greater levels of UV output and PI concentrations ultimately produce diminishing returns and even can inhibit cure.
Curing silicone-release coatings
Historically, silicone-release coatings have been developed using specially designed surface-cure photoinitiators, which rely on short UVC wavelengths emitted from broadband mercury lamps. Silicone-release coatings are among the thinnest laydowns of all UV-curing formulations, with typical coatweights around 0.6 to 1.2 gsm. As a result, these coatings do not generally require deep penetration of UV energy, which is a notable characteristic of longer UVA and UVV wavelengths.
A UV-cured, silicone-release substrate having “high release” properties requires significant energy to pull apart from a mated pressure-sensitive adhesive (PSA) surface. Conversely, “low-release” substrates require minimal energy to separate. Because silicone additives facilitate ease of release, low-release coatings always contain more silicone. While there are situations in which high-release formulations are UV-cured in open air, oxygen inhibition significantly limits the ability to reduce release energy. As a result, most free-radical, silicone-release coatings cannot be successfully cured when directly exposed to Earth’s atmosphere.
To improve crosslinking, drive low-release properties and maintain tighter process control, release coatings routinely are cured in inert environments. While maximum permissible oxygen levels vary by formulation, coatweight and desired cure quality, concentrations in the neighborhood of 50 ppm are typical. For comparison, air at standard atmospheric pressure contains 209,000 ppm of oxygen. This means that a well-engineered inert system that is closely monitored and maintained is an essential element of both the equipment and the process.
Key control factors
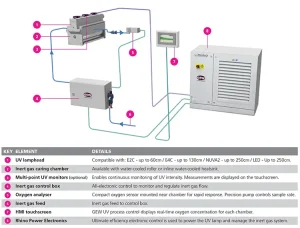
Two factors must be correctly controlled to achieve sufficient curing of a silicone-release coating. The first is the concentration of oxygen dissolved within the coating and the concentration of oxygen directly in contact with the coating surface during the time it is irradiated by UV energy. The second is the dosage, or energy density, of UVC output incident upon the release coating as it passes underneath the curing source. On converting lines, the level of atmospheric oxygen-exposed coatings is controlled using an inert chamber while the level of UV dose is controlled by one or more lampheads.
Properly designed UV-curing systems and inert chambers integrate specific controls and sensors to ensure these two factors are under control at the time of installation and throughout the industrial service life of the equipment. Typically, the inert chamber is separated from the UV lamphead assembly by a sealed quartz window. Lampheads are placed external to the chamber and arranged so the energy passes through the window and onto the coated substrate. A typical inert UV-curing system schematic is shown in Figure 1.
Inert-chamber components and operation
An inert chamber first removes the oxygen-rich boundary layer from the substrate and then sustains a low-oxygen environment. Failure to do so may inhibit the formation of free radicals as previously explained.
The boundary layer is detached from the web using angled nozzles vernacularly known as “knives.” There usually is a “knife” at the entrance of the chamber and sometimes at the exit. The nozzle design, orientation angle, distance from substrate and volumetric flowrate of the “knife” are all variables that must be carefully engineered, controlled and maintained. The “knife” is prone to contamination by the surrounding environment and must be cleaned periodically. The flowrate, distance from substrate and orientation angle must be adjusted as substrate and process conditions demand. This is because substrate thickness, surface roughness and web speed, among other variables, all have significant impact on the specific equipment setup and settings required to remove the boundary layer.
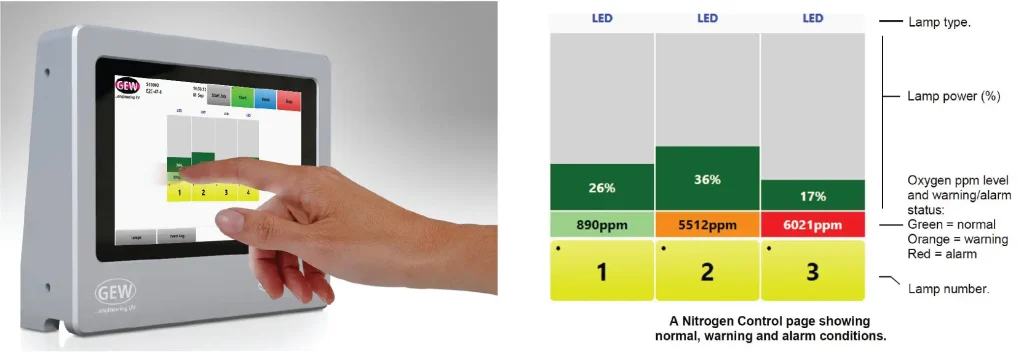
Sustaining the necessary low-oxygen environment typically is achieved by continuously flooding the chamber with an inert gas, such as nitrogen. For effective silicone-release curing, it is necessary to provide a 99.999% pure nitrogen supply. Constant monitoring and adjustment of oxygen content within the chamber allows for improved process control and a safer production environment. It also helps ensure nitrogen is not wasted.
An oxygen sensor is used as a passive measure of oxygen content within the chamber or as part of an interlocked control system (see Figure 2). An interlocked system is integrated with or without closed-loop feedback that automatically adjusts the flowrate of the inert gas to maintain the desired oxygen limit. The oxygen sensor must be kept clean, as with all parts of the chamber, but also requires periodic calibration to ensure accurate measurement. In addition, it is important to remember the position of the sampling tube typically is in the main section of the chamber and not in the boundary layer where inhibiting oxygen still is able to diffuse into the top surface of the coating.
From an engineering perspective, the removal of the boundary layer is easier to achieve if the web is supported around a roller. This allows the entrance and exit apertures and knives to be positioned very close to the web, which minimizes the inert-gas volume needed to maintain a low-oxygen environment. This is increasingly important for today’s wider coating lines.
For narrower coating lines, an unsupported web often is passed “straight through” the inert chamber as opposed to around a roller. This typically requires a larger entrance and exit aperture, making removal of the boundary layer more challenging and increasing the required volume of purging gas. Systems with “straight through” web paths often have the advantage of being equipped with a cost-effective hinging mechanism. This provides easy access to the chamber’s quartz window which, due to contamination, requires periodic cleaning.
UV-curing system
The emitted wavelengths and UV dose incident on the coating is a factor of the UV-system design, power settings, regular maintenance and web speed. Photoinitiators in silicone-release coatings typically respond best to UVC radiation; although, development activity using UVA photoinitiators is increasing. Reactivity to UVA is necessary for curing with commercially available LED systems.
Due to low coatweights, UVC dosage for effective cure of silicone release is relatively minimal compared to that required for other coatings and densely pigmented inks with thicker laydowns. An amount of 10 mJ/cm2 often is sufficient (UVC as measured using EIT PowerMap II detectors and filters.). So, it is not necessarily the power and specification of the UV lamp which is most important; it is the ability to accurately control UV output over time and with web speed, mitigate contamination and implement good maintenance practices. Both contamination and insufficient maintenance significantly alter lamp output, and faster web speeds result in reduced UV exposure. Integrated inline control methods such as UV monitoring are additional tools that are extremely beneficial for reliable lamp control.
Medium-pressure mercury arc-lamp systems are comprised of consumable parts that degrade with time and use. This ultimately results in reduced UV output relative to installation date. Additionally, inert UV systems consist of elements outside the UV lamphead, which are integral to system performance but also contribute to changes in UV output if not maintained.
Benefits of good maintenance, clean air
Arc-lamp output degrades over time due to electrode deposition as well as devitrification and solarization of the quartz tube. Degradation in UV output is not equally balanced across UVC, UVB, UVA and UVV bands. In fact, shorter UVC wavelengths deteriorate more rapidly than longer wavelengths. Furthermore, devitrification of the quartz is accelerated by contamination and humidity. Fortunately, when lamps are proactively kept clean and the system and environment are properly maintained, lamp life is prolonged.
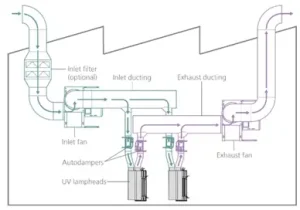
For inert systems, the lamp normally is separated from the coating by at least one quartz plate. Contaminants present in plant air can deposit on the lamp, reflectors and quartz plate whenever plant air is used to cool lamphead assemblies. Therefore, conditioning of cooling air to remove contaminants extends lamp life. This is accomplished with a “positive purge” system integrated with the lamphead assembly (see Figure 3). Additionally, the lamp itself can be cleaned periodically before eventually being replaced.
More than 70% of all UV energy emitted from electrode arc-lamp assemblies interacts with the reflector system (see Figure 6). This highlights the importance of reflector maintenance as compromised reflectors significantly reduce reflected UV energy. Photos showing new and contaminated reflectors can be seen in Figure 4. As with lamps, contaminated cooling air is the main driver for reduced reflector performance. Reflectors should be properly cooled and cleaned periodically to ensure performance. It should be noted that reflectors typically have a longer life than lamps as their deterioration is not intrinsically tied to operation.
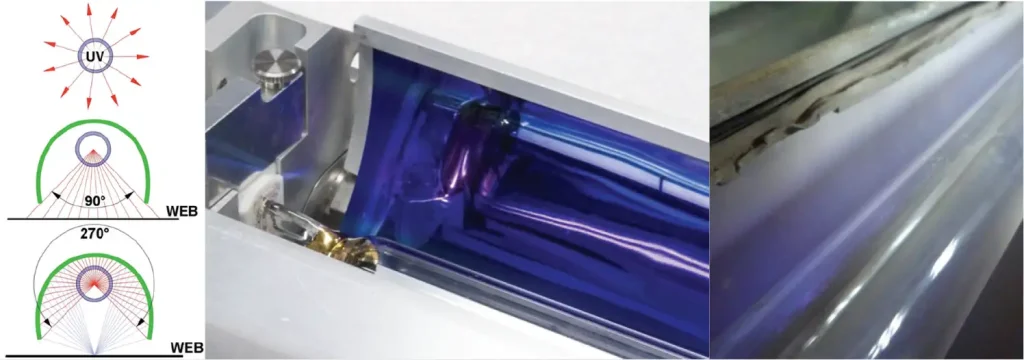
Quartz plate(s) separating the lamphead and inert chamber deteriorates in identical ways to arc lamps in terms of devitrification and solarization, leading to reduced UV transmissivity. The web-facing side of the quartz plate also is susceptible to additional contamination sources, such as coatings and substrates. Quartz plates should be cleaned periodically as instructed in maintenance manuals before eventually being replaced.
Cool off with temperature control
The UV output of a medium-pressure arc lamp is coupled with its temperature. As a result, it is prudent to appreciate that changes in lamp cooling can impact performance. Cooling issues normally manifest as undercooled systems. In other words, lamp temperatures increase, which reduces UV output. Elevated temperatures cause arc lamps to operate outside their ideal window. In severe cases, lamps will bend, sag or bulge, which further reduces UV output. High temperatures also cause changes in reflector behavior as dichroic coatings, designed to absorb infrared energy, delaminate and deteriorate.
For air-cooled systems, ducts often become blocked internally with contamination or are obstructed by ductwork collapses, severe bends and too small spaces. Installations using conditioned air can experience issues when clogged filters decrease flowrates. UV systems with combined air and water cooling usually are more robust. Commonly experienced cooling issues, such as duct movement or partial blockage in air-cooled systems, can be overcome in water-cooled systems by increasing the cooling duty of the water. Nevertheless, water systems must be regularly maintained. As a precaution, properly engineered UV systems are designed to monitor lamp temperature and power to ensure consistent operation at appropriate levels.
Checking on UV energy incidence
Despite adherence to good and regular maintenance, there is no substitute for monitoring UV energy incident upon the coating. Doing so removes all uncertainty associated with lamp output. Commonly used offline tools include color-changing, dose-monitoring “strips” and UV radiometers that periodically compare lamp output to an established UV-calibration source. Unfortunately, dose strips respond primarily to UVA wavelengths, which render them a relative measurement tool only, and radiometers are physical devices with bulky form factors that make it impossible to pass them through UV assemblies or transport them with the web.
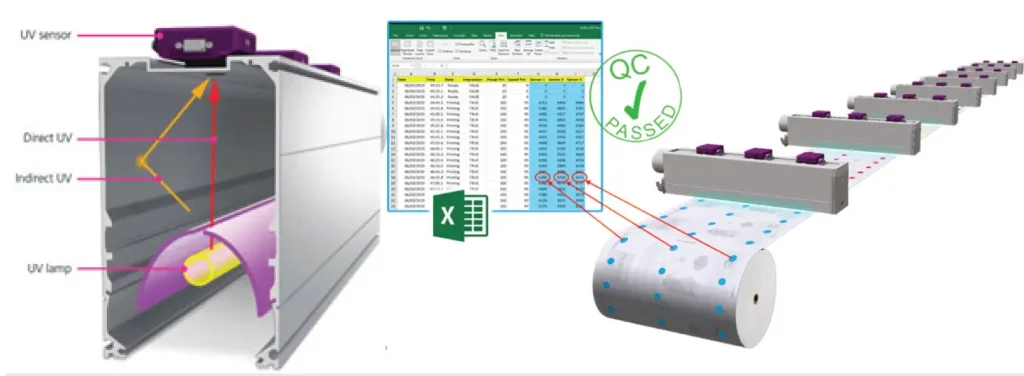
The best practice available today is to use inline UV monitors to continuously measure UV output at given positions along lamp lengths (see Figure 5). One or multiple sensors can be placed along a single lamp to record output at the edge, center or in between locations. Integrated electronic systems monitor UV-output levels and adjust system power accordingly to ensure consistent dose levels are delivered to silicone-release coatings at all times.
Conclusion
Curing silicone-release coatings with ultraviolet energy is established technology. It enables narrow-, mid- and wide-web converters to rapidly set coatings in small footprints at high web speeds while also producing performance properties superior to what is achievable with alternative processing methods. This transformation occurs within a fraction of a second, provided UV formulations are exposed to correct levels of UVC-energy density (dose) inside inert chambers. Inert chambers reduce oxygen concentrations at coating surfaces to prevent molecular interference within the chemical-crosslinking reaction and enable the production of low-release materials.
Once cured, coatings exit UV-curing stations and the web is immediately ready for further processing, sheeting, slitting, rewinding and shipping. Proper engineering design, maintenance and monitoring of UV-curing and inert-chamber systems are essential for ensuring long life and consistent cure of release coatings over time. All of this keeps work-in-progress goods out of inventory, reduces scrap and facilitates quicker lead times.
Reprinted with permission from Converting Quarterly (www.convertingquarterly.com).
Jennifer Heathcote, business development mgr. at GEW, Inc. (North Royalton, OH), holds a Bachelor of Science in Mechanical Engineering from Purdue University and an MBA from the Fisher College of Business at The Ohio State University. She can be reached at 440.381.5606, email: jheathcote@gewuv.com, www.gewuv.com.
Robert Rae, managing dir.-Sales for GEW (EC) Limited, holds a Master of Chemistry from Durham University. Ryan Turner, an R&D engineer for GEW, holds a Master of Engineering in Mechanical Engineering from the University of Exeter. Both are based at the GEW headquarters in Crawley, West Sussex, UK. They can be reached at +44-1737.824.500, sales@gewuv.com.