By Darren Lumber, Andreas Mock, Troy Becker, Michael Gould and Sean Des Roches, Rahn USA Corporation
In recent years, two identifiable and consistent trends have become a primary focus for the energy-curing industry: development of high-performance materials for 3D printing/additive manufacturing and increasing the use of sustainable raw materials in manufacturing to further decrease the environmental burden of a technology already lauded for being more sustainable than other traditional thermosetting technologies.
Additive manufacturing (AM) – 3D printing on an industrial scale – involves the fabrication of parts through sequential deposition of thin layers of engineering materials along a well-defined (micron-level), computer-generated pathway. These materials include metals, thermoplastics and photopolymer formulations which are applied via several possible methods, including dispensing, jetting, photocuring, laminating, spraying or thermal fusion. AM contrasts with “subtractive manufacturing,” where a finished part or product is produced from cutting, machining or shaping a product from a larger piece of material. Subtractive manufacturing creates waste from the starting raw form, which must then be removed and disposed.
The authors’ research and development focused on photocuring of thermosetting formulations in VAT polymerization processes that utilize UV or near-UV sources, including material cured via digital light projector (DLP); material cured by laser (SLA); and material cured using a projector screen (LCD).
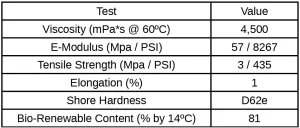
VAT polymerization involves curing of a printed image in a layer-by-layer process using a UV source generated from the bottom of a resin tank containing liquid photopolymerizable resin. A metal build plate is lowered into this resin-filled transparent tank, leaving only a very thin film of resin between the plate and the bottom of the tank. This thin film (layer) is irradiated from a UV source located underneath the tank, and the image is cured layer by layer, slowly rising from the resin tank until the entire object is printed and sits above the resin tank attached to the build plate. The source either is a laser (SLA) or projector (DLP/LCD) emitting a wavelength traditionally of 395 nm or 405 nm. The object then is removed from the build platform, washed with isopropyl alcohol and usually post-cured with UV radiation and often heat as well.
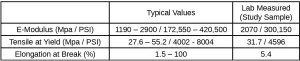
Photopolymer additive manufacturing has emerged over a 50-year period, having been employed in the past most notably for prototyping. Intricate 3D printed parts can be produced quickly at low cost compared to many traditional manufacturing techniques (e.g., reaction injection molding) to create a part or concept for manufacturers or end-users. Prototypes generally do not require substantial mechanical properties and are not designed for long-term durability, allowing for fast formulating with traditional photopolymer raw materials.
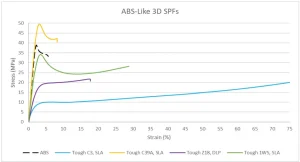
In the past decade, additive manufacturing has evolved beyond the 3D printing of prototypes to create batch runs of actual workable, durable parts. Generating products this way eventually will supplant slower and more costly manufacturing processes, particularly because photopolymer printing technology lends itself well to smaller, inexpensive production runs. It is perfectly suited to printing a limited number of products successfully, rather than thousands of pieces. However, to unseat other established technologies, printed parts need to possess mechanical properties such as strength, toughness and durability that will keep them operational for long periods of time. This places an emphasis on both formulating and developing new products to achieve these requirements.
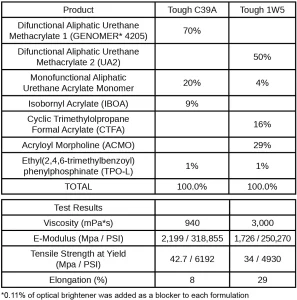
Sustainability is a second major trend among raw material manufacturers and brand owners. Both are under increasing pressure to reduce waste, limit single-source plastics from the marketplace and develop “bio-renewable” plastics as alternatives to oil-based products. Bio-based materials can offer a superior environmental profile, acting to reduce carbon footprint through renewability and reducing dependence on crude oil.
There is a “beauty” in blending 3D printing with sustainable raw materials because additive manufacturing already is regarded as a sustainable technology since the layer-by-layer building process results in less waste than in machining or diecutting. In addition, 3D printers only create the parts that are needed, resulting in less energy invested to produce finished, low-waste parts. If the raw materials involved in creating those finished printed parts are eco-friendly, the overall sustainability of the technology is increased even further.
Unfortunately, traditional sustainable energy-curable raw materials have lacked strong mechanical performance attributes. Looking to change this weak performance narrative, newly created sustainable products work quite effectively when considering three particular applications, which the authors examine and discuss in this paper.
Lost Wax Casting
Lost wax casting is a historical process for producing jewelry and models wherein an object is created from wax and coated in gypsum plaster. The wax then is melted away to leave behind a plaster mold, which subsequently is filled with molten metal to produce the desired metal item upon cooling. Reworking this process with photopolymer 3D printing creates some unique challenges. The mold itself easily can be 3D printed, which is a major benefit compared to creating it in two steps from wax and plaster. However, the thermal expansion coefficient of the photopolymer resin must be low and, being a crosslinked object, the “melting out” portion of the process may require higher temperatures than wax. Optimized burnout performance is essential, with no ash or oils left behind. All of these criteria are necessary to create a successful finished item.
One property that typically is not targeted with photopolymer chemistry, but which fits in perfectly vs. the lost wax process, is thermoplasticity. A newly developed 81% bio-renewable content monofunctional aliphatic polyester acrylate (PA1) is an exceptional product for such an application. It is a low-yellowing, high-clarity product with thermoplastic behavior that has a melting point below 100º C. It also exhibits low thermal expansion and 3D prints with very good image definition. PA1 offers the mechanical properties listed in Table 1.
This resin formulation was 3D-printable with good definition, and it maintained a melting point temperature below 100º C. The cured printed mold becomes liquid at 80º C and easily flows away from any detailed part, while requiring lower energy during removal than a typical wax. This, coupled with the fact that the new product is bio-based, drives the lost wax casting process toward greater sustainability.
ABS-Like Performance
The second application that the authors examined is considerably more demanding than the previous – a formulation with mechanical performance comparable to usable 3D-printed ABS (Acrylonitrile Butadiene Styrene). ABS is a thermoplastic polymer with a wide range of properties depending upon the specific grade, but which consistently exhibits high hardness and rigidity; good impact resistance at low temperatures; mechanical strength; toughness; low creep; great dimensional stability; good “thermoplastic weldability”; and good resistance to weak acids and alkalis. While typically exhibiting these attributes, actual mechanical properties vary widely by ABS grade, as exemplified in Table 2.
“ABS-like performance” is a common target for which much of that performance spectrum can be realized with photopolymer raw materials, just short of “high-end” ABS properties. And while ABS offers some performance advantages over typical photopolymer resin formulations, there also are features that make the polymer imperfect. Being thermoplastic, ABS typically exhibits only moderate heat resistance and, while having the ability to be printed in 3D Fusion Deposition Modeling (FDM) printers, ABS cannot be used in SLA or DLP 3D printers due to its high molecular weight and poor solubility in reactive diluent monomers. These deficiencies lead to the need for an “ABS-like” photopolymer 3D resin with low viscosity and good print capabilities. To achieve that goal, the authors’ lab focused upon developing a 3D-printable resin highlighting the impact strength of ABS.
When considering matching mechanical properties such as impact strength, knowledge of the stress-strain curves of the resin and standards became very important. The authors began by directly comparing the stress-strain curves of an ABS sample to several tough, 3D-printed formulations that had been developed in the authors’ applications lab. In Table 3, the dotted black line represents ABS, while the yellow and green formulations represent very similarly performing thermoset systems. These two SLA 3D printable formulations are detailed in Table 4 and exhibit the properties as shown.
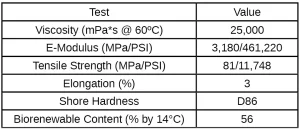
The Tough C39A formulation offers a good balance of stiffness and strength without using overly hard or highly functional raw materials, which helps to minimize shrinkage. In addition, this resin formulation exhibits low elongation with moderate tensile strength. The product was formulated with the target end use of a printed tool grip handle, which can withstand heavy vibration without deforming or loosening.
The Tough 1W5 formulation shows the best performance achieved in the authors’ lab matching the overall performance of ABS, as its mechanical values reside in the lower end of the ABS property spectrum. 3D-printed parts using this formulation provide strength with enough flexibility to produce tough, difficult-to-break pieces. Optimizing this formulation to enable increased elongation while maintaining tensile strength would result in the resin advancing from low-end to mid-range ABS properties.
To bring sustainability to this application, a newly developed product needs to be introduced. Generically referred to as UA3, this product is a difunctional aliphatic urethane acrylate with 56% bio-renewable content. It offers high clarity, thermoplastic-like behavior, shape-memory effect, good scratch resistance, low yellowing, and excellent observable light transmission. It also has exhibited very good print definition in 3D photopolymer formulations. The properties of UA3 are listed in Table 5.

In a secondary study to determine formulated property potential, the UA3 bio-based resin replaced the UA2 non-bio-based resin in the Tough 1W5 formulation at both 50 and 100% levels. The resulting mechanical testing showed UA3 increasing values in strength, toughness, and E-Modulus, and attaining ABS-like properties. The elongation was lower, but the product was tested as a drop-in and the formulation was not optimized for UA3. The results are shown in Table 6.
Lenses and Light Guides
The third application involves the properties required to 3D print lenses and light guides. The performance of such a printed part requires hardness, transparency, low yellowing and good light transmission. Each of these desired attributes lends itself very nicely to UA3. The clarity and light transmission performance is excellent and a formulation created 3D prints very good detail and really stands out in this type of application. We believe that this represents another great opportunity for UA3 as a sustainable and performance driven product.
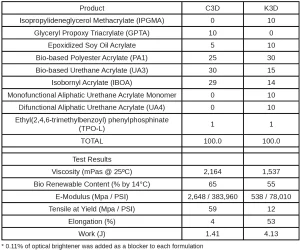
Currently, the study has progressed to developing 3D-printable photopolymer resin systems that target maximum bio-renewable content while achieving high performance mechanical properties. That work has led to the following formulations, one hard and one flexible, that maximize high sustainable content with workable performance. The formulations and test results are shown in Table 7.
3D printing, while offering a level of sustainability due to its inherent process of reduced waste, can potentially offer even greater sustainability. Newly developed bio-based oligomers consistently outperform traditional bio-based materials and offer excellent performance and practical options involving several 3D end-use applications. While only two such products are discussed in this paper, there are more products in development which also have the potential to expand the breadth of bio-based products and their inherent performance attributes. The future is bright for blending sustainability with photopolymer 3D printing!
References
- RadTech Europe Presentation – High Performance 3DP Utilizing New Sustainable Urethane (Meth)Acrylates; RAHN Group, Darren Lumber, 2021.
- Lab Report – 3D Printing; RAHN Group, November, 2019.
DISCLAIMER We have obtained this information wholly or partially from suppliers and from other sources. As we have no insight into the manufacturing processes and application procedures, the recommendations and suggestions are offered without obligation or guarantee. The customer must determine and test for himself the suitability of the products for its purpose and the substances present or not present in the goods. The details are thus conveyed with every reservation and shall imply no commitment or liability on the authors’ part. There will be no legitimate claim for any processing-/consequential damage nor responsibility regarding patent infringements. If the material is a development product, we cannot guarantee at this stage a continuous commercial supply and/or a consistent quality, unless specifically agreed upon.
For more information, visit www.rahn-group.com.