By Samantha Leap, research scientist, Chemence, Inc.
Continuous glucose monitors (CGMs) quickly are gaining popularity with an estimated 2 million patients due to their ease of use. This two-week wear[able] device allows patients to automatically check glucose levels via their phone without the need to prick their fingers multiple times a day. While this technology has been revolutionary for diabetes patients, the new device doesn’t come without issue. Skin irritation issues have been reported by patients wearing such devices caused from the UV adhesive used within the components of these devices. In fact, this issue is not seen in just CGM devices, but rather encompasses a much broader range of UV adhesives utilized in a variety of wearable devices.
Developing safer, more biocompatible, less hazardous UV adhesives will immensely help users of all wearable devices. This research dives into the development of safer, less hazardous UV adhesives by utilizing safer monomers and oligomers when formulating and ultimately ensuring these adhesives have less extractable and leachable unreacted materials. Testing via high performance liquid chromatography (HPLC) and gas chromatography-mass spectrometry (GC-MS) yielded a 93% reduction in extractable materials when compared to a low-viscosity, grade 2 ISO-10993-5 cytotoxicity-certified, UV-curable formulation utilized in needing-bonding applications. This discussion comprises formulating strategies of both traditional acrylate adhesives and the benefits of adding in a thiol:ene as a reactive diluent. The addition of a thiol:ene as a reactive diluent yielded up to a 60% reduction in extractables by means HPLC and GSMS when compared to its pure acrylate counterpart. Adhesive requirements and their appropriate test methods, biocompatibility and the future uses of low extractable UV adhesives also will be discussed at length.
Scope
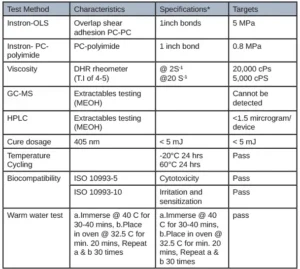
Companies seeking solutions for biocompatible UV adhesives require said adhesives to bind to a variety of different substrates as these adhesives will be utilized to adhere several different internal working pieces together. These substrates could include but are not limited to, polycarbonate (PC), polypropylene (PP), cyclic olefin polymers (COP), polyimide (PI) and even nickel (the batteries of these devices). COP has been gaining popularity in recent years in the medical device industry due to its chemical, heat and moisture resistance, high optical clarity and ability to sterilize well. Other notable common requirements of these devices are water and chemical resistance as wearable devices, especially CGMs, are worn continuously and need to withstand showers and chemicals, such as sunscreen. Viscosity of these adhesives also can vary widely depending on application. Some require low viscosities to easily wick into very small parts, while others require higher thixotropic materials so they easily can be dispensed or jetted on a manufacturing line. Material properties and glass transition temperature also widely vary depending on the type of device and application. Adhesives for wearable devices must pass rigorous biocompatibility testing by means of skin irritation and sensitization testing. Many companies are requesting adhesives be free of isobornyl acrylate (IBOA) and dimethyl acrylamide(DMA). In this paper, a biocompatible adhesive for CGMs will be discussed.
Formulating, Testing and Results
Case I: Adhesive for CGM
The first adhesive was developed for adhering several internal components together of a CGM. Table 1 illustrates the customer requirements of this adhesive and the appropriate corresponding test method.
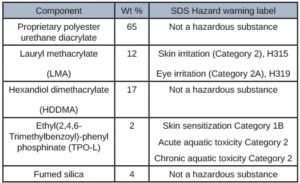
The initial formulating process consisted of investigating all the raw material SDS as well as investigating the ECHA website and avoiding raw materials that specifically had skin sensitization listed. It also was given in the scope of work that IBOA and DMA could not be used. Based on this information, methacrylates became the focus as these typically are less hazardous raw materials. After many iterations of formulations with methacrylate oligomers, it was clear that a different direction was needed because the adhesion targets were not being met. A high molecular weight polyester urethane diacrylate with no hazard labels listed on the SDS was formulated in combination with several methacrylate diluents. This formulation showed promising adhesion results as well as extractable results. TPO-L was used as the photoinitiator as TPO solid now is banned in several countries due to its high toxicity. Using the perfect amount of photoinitiator was crucial because too little and the formulation wouldn’t cure entirely leading to excess extractables and an excess of photoinitiator would lead to high levels of TPO-l in the extractables because it wasn’t all being consumed during the curing process. Adhesion to PC and Polyimide were tested via overlap shear method and tested on an Instron. Viscosity was tested using a rheometer at several different shear rates to determine dispensability. Table 2 depicts the formulation that yielded the most promising results.
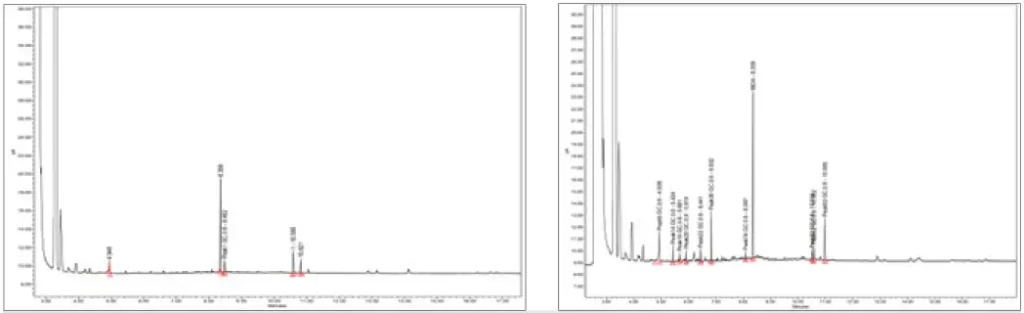
This formulation was tested for extractables by curing an open-faced film with a 405 nm LED with 5 mJ of power and then placed in 30 ml of methanol for 24 hours at room temperature. After 24 hours, the film was removed, and the methanol was run through GC-MS and HPLC for extractables. Figure 1 shows the GC-MS graph of a low viscosity, grade 2 ISO-10993-5 cytotoxicity-certified, UV-curable formulation utilized in needing bonding applications compared with the low extractable formulation. Total peak area for the standard UV adhesive was 17,612,842, while the low extractable adhesive had a peak area of 1,244,928 indicating a 93% reduction in extractables. Figures 1A and 1B illustrate the clear reduction in extractables given the lower quantity and smaller size of the peaks.
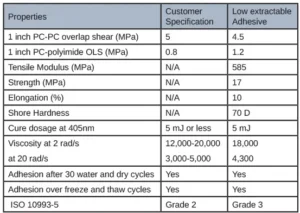
Several open-faced films were cured in the same manner and sent for cytotoxicity testing. While these films did well in this firm’s extractable testing, there still was a layer of tackiness on the top due to oxygen inhibition. Adhesives must pass this Cytotoxicity testing with a grade 2 or below to be used in a medical device adhesive. Results for the low extractable adhesive yielded a grade 3 for the ISO 10993-5 testing, indicating it was not safe for use in a medical device. Table 3 summarizes the final material properties of the low extractable adhesive.
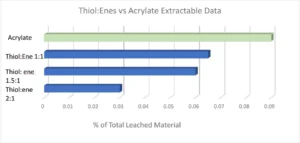
With every requirement being met except for the cytotoxicity testing, it was evident subsequent work would focus on minimizing the oxygen inhibition layer to further reduce extractables, with the expectation this would lower the cytotoxicity of the adhesives. It is fairly well known that adding a thiol:ene into an acrylate/methacrylate system is advantageous due to its improved polymerization kinetics and overall conversion. This chemistry has been utilized in the dental industry for dental restorative materials for many years. While the material properties of dental restoratives inherently are quite different from a CGM adhesive, the overall idea and chemistry of adding in a thiol:ene as a reactive diluent remains the same. In formulations where a thiol:ene is added, the thiol is consumed in the reaction by both the (meth)acrylate and the ene. It is because of this the optimum thiol:ene stoichiometry ratio deviates from a 1:1 up to a 3:1 thiol to ene ratio. Pentaerythritol tetra(3-mercaptopropionate) (PETMP) and triallyl-1,3,5-triazine-2,4,6-(1H,3H,5H)-trione (TATATO) were chosen as the thiol and ene components in this formulation. The thiol:ene ratio was varied from a 1:1 to a 1:2 and a 1:3 ratio, with the thiol:ene being in the formulation anywhere from 20-50%. Extractables were tested using the same method as previously stated. The graph below shows the percentage of total leached material by means of HPLC testing. It was evident that as thiol:ene ratio was increased there was a significant decrease in measurable extractables (Figure 2).
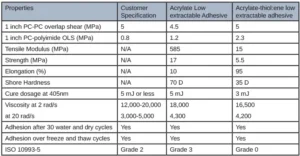
A 2:1 thiol:ene ratio decreased the extractables in the formulation by 60%; however, material properties and adhesion to polycarbonate and polyimide still had to be considered. Ultimately, a 1.5:1 thiol:ene ratio at 30 weight percent into the low extractable adhesive was concluded due to all the customer requirements being met. A 1.5:1 thiol:ene ratio still had a reduction in extractables by almost 35%. Open-faced films were cured with a 405 nm light with 5 mJ of power and sent for cytotoxicity ISO 10993-5 testing. These films received a grade 0 (meaning 0% cell death occurred) cytotoxicity rating, which is rare from a UV-curable material as most UV adhesives are grades 1 and 2. The odor of the adhesive was not an issue due to the high functionality and high molecular weight thiol chosen. Low-odor versions of thiols are commercially available; however, some exhibit stability issues in comparison with their regular odor counterpart. Since the customer was using a very small amount of adhesive applied via a syringe (closed system), the smell on the manufacturing line and in the final device would be negligible. It also is worth noting that, depending on formulation, cure conditions and normal experimental deviation, the photoinitiator, TPO-L contributed anywhere from 10-70% of the extractables due to the TPO-L not incorporating into the polymeric network. Since thiol:enes are more reactive (and therefore less photoinitiator was used), less TPO-L was seen in the extractables. Material properties of the new thiol:ene low extractable formulation can be seen in Table 4 in comparison with the acrylate low extractable formulation.
UV-curable acrylate-thiol:ene adhesives have the potential to significantly improve adverse skin irritation and sensitization issues seen by users of all wearable devices but especially those who must wear continuous glucose monitors. The reduction of extractables when adding in a thiol:ene as a reactive diluent was seen to be between 30-60% ,which ultimately led to a ISO10933-5 grade 0 non-toxic UV curable adhesive.
A second case (see below) dives into the development of a cyclic olefin polymer UV adhesive for use in an electronic cigarette application.
Conclusion
Wearable devices have been gaining popularity in the last decade, from Fitbits and Apple watches to wireless earbuds and continuous glucose monitors. It has been noted that end users have skin irritation and sensitization from the adhesives used within these devices leaching out over time. This especially is true in continuous glucose monitors as these are never taken off patients. Developing safer, more biocompatible adhesives for wearable devices is essential in today’s UV curing market. More and more customers are requiring adhesives to be isobornyl acrylate- (IBOA) and dimethyl acrylamide- (DMA) (known sensitizers) free. When formulating such adhesives, it’s crucial to be cognizant of the raw material’s hazardous and sensitization status. Starting the formulating process from safer raw materials is a surefire method to ensure a safer cured material. Ensuring extractables and leachable materials are being tested and are well below safe thresholds also is pivotal. Extractables and leachable can be minimized by making sure the adhesives are curing well and even adding in a thiol:ene as a reactive diluent to the formulation. Thiol:enes has been known to be advantageous due to their improved polymerization kinetics and overall conversion. This chemistry has been utilized in the dental industry for dental restorative materials for many years. This knowledge easily can be applied and modified to obtain different material properties and adhesion characteristics based on specific adhesive projects and customer requirements.
A market gap exists for low surface energy UV adhesives and, even more specifically, biocompatible low surface energy UV-curable adhesives as more companies are switching to low surface energy plastics for their wearable devices. The low-extractable, low-surface-energy polypropylene adhesive was formulated to be hydrophobic, exhibit an extremely low surface energy while still offering robust material properties, good adhesion performance and, most importantly, superior biocompatibility. While this adhesive was developed for an electronic cigarette application, the learnings can certainly be utilized in the future on other biocompatible wearable device adhesives.
References
- Sirish K. Reddy, Neil B. Cramer, Christopher N. Bowman. Thiol−Vinyl Mechanisms. 1. Termination and Propagation Kinetics in Thiol−Ene Photopolymerizations, https://pubs.acs.org/doi/full/10.1021/ma060249m
- Sirish K. Reddy, Neil B. Cramer, Christopher N. Bowman. Thiol−Vinyl Mechanisms. 2. Kinetic Modeling of Ternary Thiol−Vinyl Photopolymerizations, https://pubs.acs.org/doi/full/10.1021/ma0600097
- Neil Cramer, Charles L. Couch, Kathleen M. Schreck, Jordan E. Boulden, Robert Wydra, Jeffrey W. Stansbury, Christopher N. Bowman. Properties of methacrylate-thiol-ene as dental restorative materials. Properties of methacrylate–thiol–ene formulations as dental restorative materials – ScienceDirect, https://pubmed.ncbi.nlm.nih.gov/20553973/
- Ensuring Adhesion for Medical Device Assembly | 2019-03-12 | New Adhesives for Medical Device Assembly | 2022-03-04 | ASSEMBLY (assemblymag.com)
Samantha Leap is a research scientist for Chemence, Inc., Alpharetta, Georgia. She received her chemical and biochemical engineering degree from the Colorado School of Mines. She started her career in the UV polymers industry in 2013 at Colorado Photopolymers Solutions. She has 10 years of experience working in the UV coatings and adhesives industry and now works for Chemence specializing in UV curable adhesives for electronic and medical devices. For more information, email sleap@chemence.com or visit http://chemence.com.
Case II: UV adhesive for electronic cigarette
UV adhesives are not readily utilized in bonding of polyolefins due to their low surface energy. The polyolefin family consists of cyclic olefin copolymer (COC), polyethylene (PE), and polypropylene (PP). Polypropylene is advantageous due to its lighter weight, better flexibility, lower cost and overall, less harmful chemicals. Offering a biocompatible low surface energy UV adhesive would not only save companies time and money on manufacturing costs over traditional adhesives but more importantly, it is much safer for the consumer.
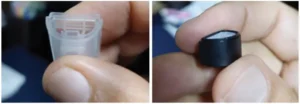
An electronic cigarette is probably not the first device that comes to mind when asked about a wearable device. While an electronic cigarette is not a fully wearable device, the requirements around making an adhesive for its assembly is extremely similar to that of a wearable device. The adhesive must meet rigorous biocompatibility requirements as part of the cigarette goes into someone’s mouth and is constantly being held in their hand. The customer requested an IBOA and DMA free adhesive for polypropylene. These were the extent of the customer requirements that were provided. Below are several pictures to illustrate where the adhesive was being applied.
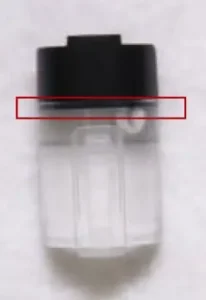
After a small amount of adhesive was dispensed into the polypropylene body, the top and rubber gasket were placed, the adhesive was cured using a 405nm LED. Below is a picture of the constructed electronic cigarette body.
Formulation development consisted of utilizing a plethora of commercially available monomers, oligomers, photoinitiations and surface additives. A hydrophobic urethane acrylate was selected as the best oligomer, as were several diluents with low surface energy. The formulation surface energy was further lowered by adding a silicone wetting agent and finally a photoinitiator was selected to cure at 405 nm wavelength LED. It was determined that the adhesive viscosity needed to be extremely low in order to wick into the small narrow bond line. The adhesive’s final material properties were formulated so that the adhesive was both a strong and flexible formulation making it an extremely robust adhesive material. Table 5 shows the formulation.
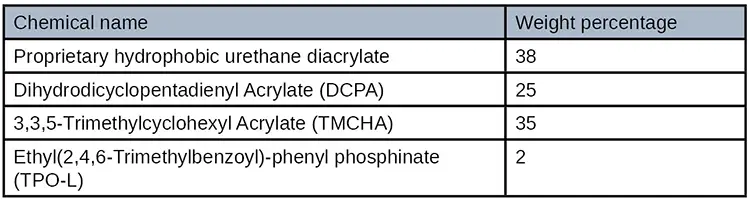
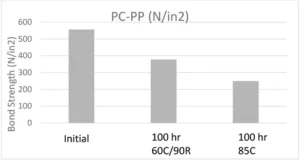
Numerous tests were performed on this adhesive including overlap shear adhesion, extractable testing, tensile material properties, and finally biocompatibility. Adhesion tests were done by means of overlap shear testing and extraction testing was performed on both HPLC and GC-MS. Adhesive films were cured between two glass slides using a broadband light and tested for cytotoxicity and skin sensitization (ISO 10993-5 ). One-inch overlap adhesive bonds were made and pulled with an Instron in accordance with ASTM D1002. Initial adhesion results were measured as well as bond strength after aging the bonds for 100 hours in 60˚C 90% relative humidity and 85˚C conditions. Below is the graph showing adhesion results for PC-PP bonds.
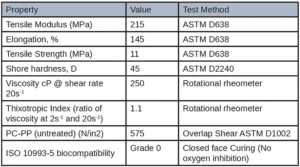
Although there was a drop in adhesion after heat and humidity aging (this is to be expected), the adhesive retained enough adhesion to be accepted by the customer.
Extractable testing was performed on all cured samples. Samples were cured in between two glass slides with a broadband light and then left to sit in methanol for 24 hours at 25˚ C. The sample was then taken out of the methanol and the methanol was run through HPLC and GC-MS to test for any unreacted monomers in the formulation. Results of the extraction testing yielded promising results especially when compared to other commercially available UV adhesives. The figure below shows the GC-MS extractable data of a standard UV needle bonding adhesive and the low extractable polypropylene adhesive developed for this application. The standard UV adhesive has a peak area of 17,612,824 while the low extractable polypropylene adhesive had a peak area of 1,107,985 yielding again a 93% reduction in extractables. These favorable results can be seen on the GC-MS overlay below by the smaller and overall, less quantity of peaks. All other material properties were tested and can be seen in Table 6.
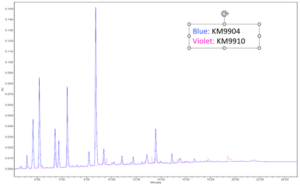
The tensile properties and adhesion targets were deemed to be well performing based on the customer’s drop tests and pull apart test of the electronic cigarette body. A high elongation material was needed to withstand drop tests while the moderate tensile strength and modulus were needed to withstand several rounds of attempting to be pulled apart. This adhesive also passed a glycol resistance test which was a proprietary test conducted by the customer. A grade 0 was reported for ISO 10993-5 biocompatibility indicating 0% cell death occurred when tested. This adhesive film was cured between glass slides to replicate how the adhesive would be cured in the electronic cigarette top and body before being sent for cytotoxicity testing. Oxygen inhibition was thus not an issue for this application. The cytotoxicity results are promising in that, if there is any unreacted leachable material in the adhesive, it would not be harmful to the end user.