By Igor V. Khudyakov, PhD, DSc, Research and Development Director, Performance Coatings International Laboratories
Introduction
Urethane acrylates (methacrylates) are valuable oligomers widely used in industry. They are used in adhesives, protective and decorative coatings, dental fillings, labels, inks and as coatings for optical fiber. As review, synthesis of the most common and simple urethane acrylates consists of two steps and includes three reagents: polyol-diol (P) polyether or polyester, diisocyanate (D) and a capping agent – hydroxyl-substituted acrylate (A). The most common diisocynates are isophorone diisocyanate (IPDI), tolylene diisocyanate (TDI), methylene diphenyl diisocyanate (MDI) and p-phenylene diisocyanate (PPDI). The acrylate often is 2-hydroxyethyl acrylate (HEA) or 2–hydroxyethyl methacrylate (HEMA).
In the first step, the polyol-diol reacts with an excess of the diisocyanate. After that the oligomer D–P is capped with the acrylate (the second step). Elevated temperatures and catalysts are required in each of the two steps. The final oligomer should result in all reagents having reacted with each other. The number of used equivalents (n) of NCO of the diisocyanate will be equal to the sum of OH equivalents of the polyol-diol and the acrylate:
Equation 1. n(diisocyanate) = n(polyol-diol) + n(acrylate)
or
n(D) = n(P) + n(A)
Completion of a reaction is usually controlled by infrared (IR). Fortunately, the NCO group has strong absorption in the mid-IR spectrum (2270 cm-1)1,2, and it allows monitoring of the disappearance of NCO with high accuracy. Selection of one or another diisocynate, polyol-dio, acrylate and a molecular weight of polyol-diols allows the creation of urethane acrylates with a wide range of physical/mechanical properties in the cured and the liquid state. Further, a ratio of ingredients n(D): n(P): n(A) can be varied. Such variation obviously leads to the changes in properties of the final product. Certainly, variation of n(D): n(P): n(A) should not violate Equation 1.
The method described above often is called direct synthesis.1 It is possible to run a reverse synthesis.1 In reverse synthesis, the first step is a reaction between the diisocyanate and the acrylate, with subsequent reaction of D–A with the polyol-diol. Interestingly, the same amounts of the same diisocyanate, polyol-diol and acrylate lead to products with quite different properties prepared by the direct or reverse synthesis. Reverse addition leads to lower molecular weight (MW) and lower viscosity (ƞ) of the final urethane acrylate. Direct vs. reverse synthesis is another purposeful way to change properties of the synthesized urethane acrylates with the same amount of the same reagents.1
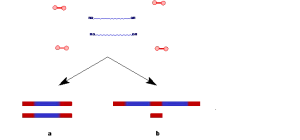
Low Free monomer (LF) oligomers. In the first stage of (direct) synthesis, chemists often take n(D) = 2 n(P). A naïve expectation is that the product D–P will have a structure as presented in Figure 1a. However, formation of the product presented in Figure 1b occurs. Macromolecules presented in Figure 1b are formed by chain extension of the primary D-P products by the second polyol-diol molecule. In a general case, both products schematically presented in Figure 1 are formed. Moreover, NCO-terminated macromolecules may include more than two polyol-diol molecules in their structures.
Products of a reaction between diisocynates with polyol-diols change under condition n(D) ≥ 10 n(P). Under such a condition, a chain extension (Figure 1b) is negligible because the polyol-diols are surrounded by a large excess of diisocynates. Rate (or probability) of a reaction of polyol-diols with diisocynates is much higher than with P–D because [D]>>[P–D]. Moreover, a rate constant of a reaction of a hydroxyl group with free diisocynates usually is higher than that of a reaction of –OH with a second –NCO of D–P.3
Thus, the product formed under the condition n(D) ≥ 10 n(P) is presented on the Figure 1a. The mixture has an excess of non-reacted diisocynate. Diisocynate can be stripped under vacuum, and an individual product (Figure 1a) is an LF oligomer or LF prepolymer. LF stands for the extremely low concentration of non-reactive hazardous free diisocynate. LF oligomers are commercially available at a modest cost. They are not hazardous: reactive–NCO is present, but it is bound to a macromolecule polyol-diol. LF oligomers are thermally cured with different curative agents [1,4- butanediol, 2-aminoethanol, 4,4’- methylenebis(2-chloroaniline) (MOCA), others] in the preforms. Wheels, belts, balls and other high–performance objects are made from the cured LF oligomers.
Obviously, LF oligomers have relatively low molecular weight distribution, equal to that of the initial polyol-diol (Figure 1a).4 Common oligomers have wider molecular weight distribution due to chain extension (Figure 1a and b). In this article, we compare common and LF oligomers, which have the same ratios n(D): n(P): n(A) = 2:1:1 in the final product. Further, LF oligomers have a lower viscosity (h) than common oligomers.4 One may argue that it is not obvious that common oligomers have higher h than the relevant LF oligomers. High h of high molecular weight chain-extended products may be compensated by low h of non-reacted diisocynate (Figure 1b). Diisocynate usually is a liquid at ambient conditions. However, for many polymers, h ~ Mk, where k > 1. That way, contribution of high MW components into h is not compensated by low MW components – at least in this case.
Capping of LF oligomers
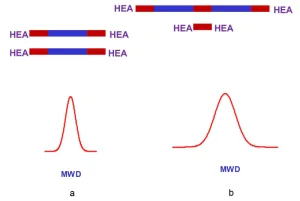
Different commercial LF oligomers can be capped with hydroxyl acrylates in order to get urethane acrylate. It is the one-step reaction mentioned in the title. The capping agent will be HEA. The molecular weight distribution of urethane acrylate made with LF oligomers will be smaller than those of analogous common urethane acrylates. (See Figure 2.) The h of common urethane acrylate is higher than that of relative urethane acrylates made with LF oligomers (cf., above). Oligomers with relatively low h are valuable because they require none (or a small amount) of reactive diluent, which changes properties of the final curable coating.
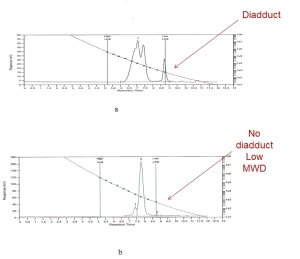
One will notice that common urethane acrylates include low molecular weight compound HEA-D-HEA (Figure 2b). Low MW additives usually have a detrimental effect on the elastic properties of the cured coatings. HEA–D–HEA are usually solid compounds under ambient conditions. Cure of specially prepared melted A–D–A compounds leads to their shatter under light or to very hard coatings. Urethane acrylates prepared from LF oligomers do not have A-D-A. The latter compound often is called diadduct. Experimental data (Figure 3) confirm the statements made above.
A summary of properties of materials prepared by direct vs. reverse synthesis,1 prepared at different D/P ratios2 and acrylated LF vs. common urethane acrylates4 is presented in the cited literature.
The possible application of LF oligomers in the coatings for optical fiber
Acrylated LF oligomers may find an application in photocurable coatings for optical fiber. The primary coating of optical fiber should be elastomeric, soft, have high elongation-to-break, low Tg, low storage (elastic) modulus and low molecular weight distribution.5 (Many of these requirements listed above are interdependent.) Low molecular weight distribution of coatings for optical fiber is required for a speedy application of coating.5 LF oligomers capped by HEA or another acrylate are promising candidates of coatings for optical fiber.
Conclusions
Photocurable urethane acrylates can be made in one stage by the catalyzed addition of hydroxyl-substituted acrylate to commercial LF oligomers. A variety of commercially available LF oligomers, as well as capping agents, allows simple synthesis urethane acrylate oligomers with different properties in the liquid and cured state. That makes LF oligomers especially valuable for small or startup companies that intend to produce cost-effective products with a wide range of applications. Additional benefits of such urethane acrylates are their relatively low viscosity and low molecular weight distribution. υ
References
- Swiderski, K.W.; Khudyakov, I.V. Industrial & Engineering Chemistry Research, 2004, 43, 6281.
- Khudyakov, I.V.; Swiderski, K.W.; Greer, R.W. Journal of Applied Polymer Science, 2006, 99, 489.
- Szycher, M. Szycher’s Handbook of Polyurethanes; CRC Press: Boca Raton, FL, 1999, ch 2.
- Zhu, Z.; Khudyakov, I.V.; Rosenberg, R.O. Radiation-Curable Polyurethane. US6943202B2.
- Khudyakov; I.V.; Purvis; M. B.; Overton, B. J. Formulation of UV-curable coatings for optical fiber for a fast cure. US 6887918.
Acknowledgment
The author is grateful to Vince Gajewski (ret.) and Jerry Bryce of COIM USA for useful discussions.
Igor V. Khudyakov, PhD, DSc, is research and development director at Performance Coatings International Laboratories, Bangor, Pennsylvania. PCI is a manufacturer and supplier of high-performance oligomers and coatings, including thermal and UV-curable coatings with high abrasion resistance. Khudyakov holds 21 US patents, has written or contributed to more than 200 scientific and technical publications, and has presented at a number of conferences, including RadTech International North America and RadTech Europe. For more information, email ivk@pcoatingsintl.com or startatj@aol.com.