By Joseph D. Lichtenhan, Hybrid Plastics Inc.
Abstract
Polyhedral oligomeric silsesquioxane (POSS®) additives provide a simple-to-use chemical tool kit that requires only small loading levels to improve traditional formulations. At 1.5 to 3.0 nm in diameter, POSS cages provide a tremendous amount of surface area and volume control when incorporated into formulations. These additives also are used as compatibilizing tie-layers in coating stacks and to impart adhesion, hardness and scratch-resistance properties in optical coatings. Fundamental attributes of methacryl POSS and glycidyl POSS additives are presented and related to material science concepts that explain the observed effects in coating formulations.
Introduction
Additives that can be utilized at low percentages to expand the performance of existing polymer systems have become a growth area within the specialty chemicals industry. In response to the demand, families of polyhedral oligomeric silsesquioxane (POSS) chemicals were developed. Each POSS cage melds the desirable thermal stability and modulus of inorganic additives (SiO1.5) with organic (R) groups to render compatibility and reactivity with heritage polymers, monomers and ingredients. For coatings, methacryl POSS and glycidyl POSS find use as additives in new and emerging formulations.
Representative structures for methacryl POSS and glycidyl POSS are shown in Figure 1. While octameric cage sizes are shown, the SiO1,5 cage core sizes are a mixture of octamer, decamer and dodecamer. The cage size distribution renders a melting point depression and allows these additives to exist as clear, low-viscosity liquids. Both of these compositions are highly miscible with nearly all conventional coating resins, oligomers and monomers.
These additives also are soluble in common organic solvents and are characterized by GPC, HPLC and NMR. A summary of the physical properties have been tabulated in Table 1.
The chemicals differ from traditional chemical additives by providing an envelope of effects in coatings. The envelope of effects is based on the composition, rigidity, surface area, volume and chemical reactivity of the additive.
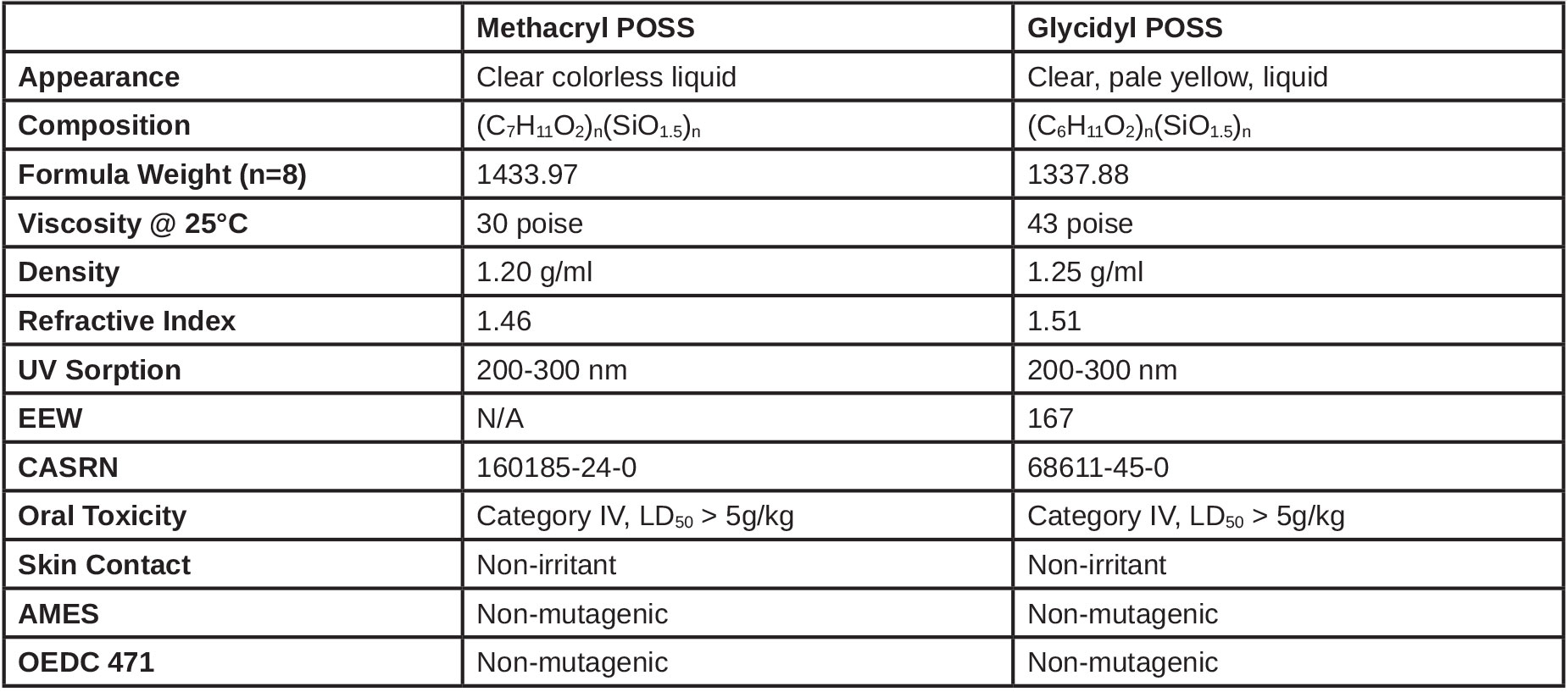
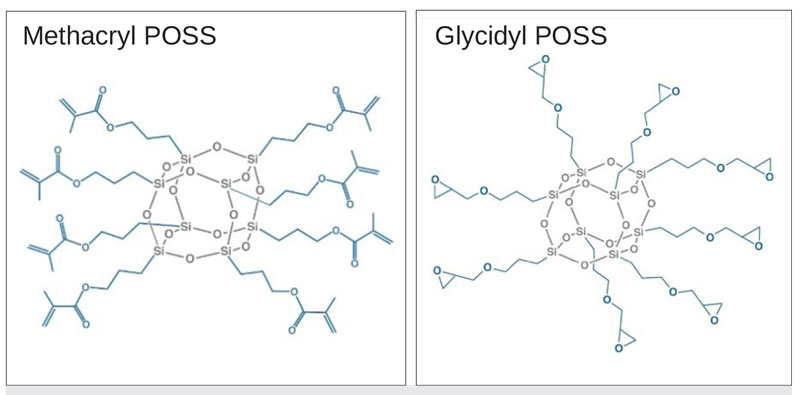
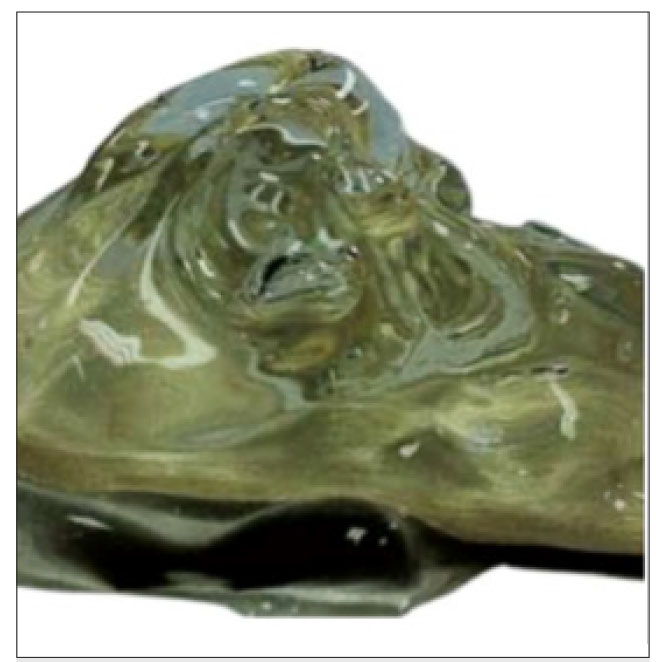
Attributes that lead to enhanced coatings
Attribute 1.
The modulus of the cage is unique and rigid at 11.7 GPa.1 The silicon-oxygen core also is mechanically2 and thermally durable3. In formulary work, the additives can impart stiffness (modulus) and hardness. Thus, it often is recommended to formulate the additives with flexible comonomers/oligomers to counter any undesirable modulus build.
Attribute 2.
The cross-linking capability of these additives leads to rapid gelation with increased modulus and hardness. When high degrees of conversion are achieved, the glass transition also will increase. However, at lower degrees of conversion, any nonreacted arms on the cages can serve as internal plasticizers. These cages do not provide the same level of hardness as wholly inorganic additives. However, they can be used synergistically with fillers to impart additional hardness, reinforcement and durability.4
Attribute 3.
The rigid core, in combination with the long reactive external arms, gives molecular diameters in the range of 1.5 to 3.0 nm. These large diameters enable the additives to provide surface area control and extensive contact with other ingredients in a formulation. In this respect, the additives often serve as compatibilizers between ingredients and dissimilar formulation components.5

Attribute 4.
The large diameters of the additives enable them to provide volume control inside of formulations. When well dispersed into polymers, these additives tend to increase the spacing between polymer chains. The increased volume provides improved rheological properties (a diluent-like effect) and also results in modest improvements in toughness and impact properties. Additionally, when associated to filler and ingredient surfaces, POSS cages improve dispersion and effectiveness.6
Attribute 5.
Finally, the hybrid organic-inorganic composition enables formulations containing it to undergo surface glassification. Surface oxidation is common in many processes as a method to provide enhanced adhesion, bonding and printability. These processes include: oxygen plasma etching, corona treatment, short wavelength UV, ozone and flame treatment. From a chemistry perspective, the oxidation process involves the removal of organic groups from the cage and subsequent fusing together of the cages (similar to sintering) to form a surface glass layer. The resulting surface glass layer is nanoscopically thin and, while not completely abrasion resistant, it does improve hardness, scuff and mar resistance. The surface glassification also can serve as an ideal bondable tie-layer between coatings.7
Examples to illustrate effects in coating formulations
Dispersion and Rheological Effects
Cage diameters range from ~1.5 to 3 nm and are less than 1/4 the diameter of a 20 nm silica particle. Methacryl POSS and glycidyl POSS associate with the surface of nanosilica and afford clear dispersions at 30 wt% nanosilica loading levels. Nanosilica, in combination with POSS, can be viewed as two reinforcing agents in one and can be readily used in the formulation of hard and durable coatings.8 Such formulations also are amenable to surface glassification, thereby providing in situ hard coatings that are suitable for use as bonding tie-layers.9
The dispersion effect of methacryl POSS and glycidyl POSS also can also be utilized synergistically with particles and ingredients containing silane coupling agents.10 The advantage of this turnkey approach is that it does not require changing from heritage coupling agents as surface modifiers, while the addition of the additives extends their performance to new levels.
Unique rheological synergies also can be realized when using the additives as dispersion agents. For example, when equal parts of glycidyl POSS and diglycidylether bisphenol-A (DGEBA) are mixed with 5 wt% of fumed silica, a rheological synergy results (Figure 3: bottom-blue trace). The synergistic combination exhibits a drastically reduced viscosity relative to the individual viscosities of 5 wt% fumed silica in diglycidyl bisphenol-A (top: red trace), or when 5 wt% silica is added to glycidyl POSS (middle: gray trace). The synergy is believed to arise from the ability of the additive to associatively bond to the surface of the silica particles and provide volume around the particles while also rendering it more compatible with the DGEBA resin. Such rheological enhancements can enable the manufacturability of highly solid-loaded formulations without the use of a solvent or oils, which degrade physical properties.
Crosslinking and mechanical effects in epoxyacrylate coating resin
Epoxyacrylate (EA) is a class of resins that blend the desirable physical properties of epoxy resins with the desirable cure characteristics of acrylics. EAs are widely utilized in UV curing systems to provide enhanced chemical resistance, adhesion and mechanical strength. A deficiency of EAs is their inability to reach complete cure (olefin conversion), due in large part to the low ratio of curable olefin relative to molecular weight. Therefore, the incorporation of these additives can be viewed as a logical solution.
A study was conducted using Sartomer CN120 (EA) in combination with loading levels of methacryl POSS up to 10 wt% relative to EA.11 The photoinitiators benzophenone (2.8 wt%) and triethanolamine (0.96 wt%) were used to effect UV cure using 15- sec. exposures from a 5 kW high-pressure mercury lamp.
The addition of the additive at 3 wt% and 10 wt% levels increased the double-bond conversion, as anticipated. The EA alone reached a plateau conversion level of 80%, while the addition of 10 wt% methacryl POSS achieved conversions approaching 95%. The enhanced conversion was attributed to the miscibility of the additive with EA, and the high volume and surface area of methacrylic groups provided relative to its molecular weight.
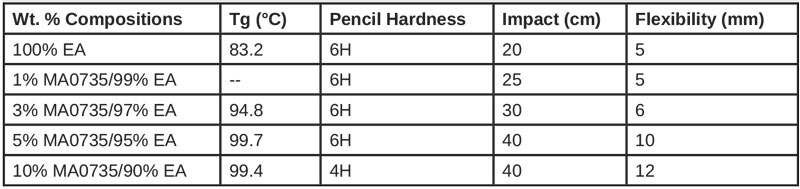
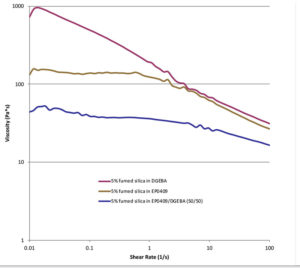
The glass transition (Tg) was observed to increase with the loading level. The addition of 10 wt% methacryl POSS resulted in a 20 to 25°C increase of Tg (via dynamic mechanical analysis), for which the tan ∂ transition was significantly broadened and its enthalpy significantly reduced. These observations are consistent with the cage cross-linking and restricting local chain-network motion at temperatures above the glass transition, thereby retaining more glassy-state characteristics at elevated temperatures.
The impact and flexural properties of the formulations were enhanced. For example, at a 5 wt% loading of the additive, the impact and flexural values were doubled relative to the EA control. Beneficially, the 5 wt% loading also maintained a pencil hardness of 6H.
The enhancement of impact and flex while simultaneously increasing cross-link density can seem counterintuitive. However, the contributions of volume and surface area may enable these additives to provide such effects in addition to its role as a cross-linker. The authors of the work also attribute the improvement of impact resistance to the ability of the additives to absorb and dissipate energy through a plastic deformation mechanism as opposed to undergoing brittle fracture. The ability of nanostructures, such as silica, to control free volume in formulations and their ability to absorb and dissipate energy is well known.12 It appears that the additive behaves in a similar manner, with the additional advantages of being miscible, cross-linkable, transparent and dilutive.
Nonreactive blending and mechanical effects in epoxyacrylate coating resin
Using the same EA resin, glycidyl POSS was incorporated at loading levels up to 10 wt% relative to EA and tripropylene glycol diacrylate (TPGDA). The TPGDA was maintained at 55.6 wt% of the formulations. Benzophenone (BP) and triethanolamine (TEA) were used as photoinitiator and co-initiator, and cure was conducted using a high-pressure mercury lamp with an intensity of 260 mW/cm2 for 15 seconds.

Because epoxy acrylate resins (EA) do not contain any oxirane groups, neither the EA nor the glycidyl POSS was not anticipated to participate in UV cure. However, the oxirane groups on glycidyl POSS and its high surface area and polarity were anticipated to improve the overall miscibility cure rate of the TPGDA through interfacial compatibilization.13
The incorporation of glycidyl POSS at only 1 wt% was observed to increase the glass transition (Tg) of the formulation to a maximal level of ~93°C. This finding indicates that, at this loading level, a restriction of chain motion was achieved, presumably through associative interactions between the cages and the EA, despite the absence of covalent cure.
The impact properties at 1 wt% loading were improved by 50%, while at 5 wt% loading levels of glycidyl POSS, the impact properties were improved by ~ 150 % relative to the control resin. This enhancement was attributed to associative interactions between the additive and the epoxy acrylate, which served to prevent the propagation of cracks and increase the dissipation of energy. Logically, the high surface area and volume at or near the additive contributed to such interactions.
Conclusions
At first glance, glycidyl and methacryl POSS can be considered as cross-linkable additives for coatings and resin systems. However, their hybrid (organic-inorganic) compositions and high density of reactive groups, in combination with surface area and volume contributions, enable these additives to provide an envelope of physical property enhancements to coatings. In particular, these systems are effective at providing dispersion, rheological control and modulus, and impact enhancement, in addition to the traditional cross-linking function. u
Acknowledgements
The author expresses gratitude to Dr. Joseph Schwab and Marion Blue of Hybrid Plastics for useful technical discussions. The careful reading and editing of this manuscript by Mike Carr also is appreciated.
References
1. Capaldi, F.M., Boyce, M. C., Rutledge, G.C. The mechanical properties of crystalline cyclopentyl polyhedral oligomeric silsesquioxane. J Chem Phys. 2006 Jun 7; 124(21):214709, DOI: 10.1063/1.2208355
2. Ionescu, T.C, Qi, F., McCabe, C., Striolo, A. Kieffer, J., Cummings, P.T. Evaluation of Force Fields for Molecular Simulation of Polyhedral Oligomeric Silsesquioxanes. J Phys Chem B. 2006, 110 (6):2502-10. DOI 10.1021/jp052707j
3. Mantz, R.A, Jones, P.F., Chaffee, K.P., Lichtenhan, J.D., Gilman, J.W., Ismail, I.M.K., Burmeister, M. J. Thermolysis of Polyhedral Oligomeric Silsesquioxane (POSS) Macromers and POSS-Siloxane Copolymers. Chem. Mater., 1996, 8 (6), 1250–1259. DOI: 10.1021/ cm950536x
4. Fong, H., Dickens, S.H., Flaim, G.M. Evaluation of dental restorative composites containing polyhedral oligomeric silsesquioxane methacrylate. Dent Mater. 2005, 21(6):520-9. DOI: 10.1016/j. dental.2004.08.003
5. Prządka, D., Andrzejewska, E., Marcinkowska, A. Multimethacryloxy- POSS as a crosslinker for hydrogel materials. European Polymer Journal 2015 (72), 34-49. https://doi.org/10.1016/j.eurpolymj.2015.09.007
6. Barra, G., Vertuccio, L., Vietri, U., Naddeo, C., Hadavinia, H., Guadagno, L. Toughening of Epoxy Adhesives by Combined Interaction of Carbon Nanotubes and Silsesquioxanes. Materials 2017 25;10(10). pii: E1131. doi:10.3390/ma10101131
7. Lee, B.K., Cha, N-G., Hong, L-Y, Kim, D-P, Tanaka, H., Lee, H.Y., Kawai, T. Photocurable Silsesquioxane-Based Formulations as Versatile Resins for Nanoimprint Lithography. Langmuir, 2010, 26 (18), 14915– 14922. DOI: 10.1021/la1025119
8. (a) https://hybridplastics.com/product/ma4l35-01-nanosilica-dispersion-methacrylate-poss/ (b) https://hybridplastics.com/product/ ep4f09-01-nanosilica-dispersion-epoxy-poss/
9. T. J. Engardio, G. Y. Hu, D. K. Kang, E. R. Rorye, US. Patent 8,163,357.
10. D’Arienzo, M., Redaelli, M., Callone, E., Conzatti, L., Di Credico, B., Dirè, S., Giannini, L., Polizzi, S., Schizzi, I., Scotti, R., Tadiellod, L., Morazzonia, F. Hybrid SiO2@POSS nanofiller: a promising reinforcing system for rubber nanocomposites. Mater. Chem. Front., 2017,1, 1441- 1452. DOI: 10.1039/c7qm00045f
11. Wang, Y., Liu, F., Xue, X., Morphology and properties of UV-curing epoxy acrylate coatings modified with methacryl- POSS. Progress in Organic Coatings 2105 (78), 404-410. http://dx.doi.org/10.1016/j. porgcoat.2014.07.003.
12. Zhenga, Y., Zheng, Y., Ning, R., Effects of nanoparticles SiO2 on the performance of nanocomposites. Materials Letters 2003 (57) 19, 2940- 44. doi:10.1016/S0167-577X(02)01401-5
13. Wang, Y., Liu, F., Xue, X., Preparation and properties of UV-cured epoxy acrylate/glycidyl-POSS coatings. Journal of Coatings Technology and Research, 2017, (14) 3, 665–72. DOI 10.1007/s11998-016-9886-1.
Joseph Lichtenhan is vice president of new business development at Hybrid Plastics Inc., Hattiesburg, Mississippi. He earned a doctorate in chemistry at the University of California, Irvine, and was involved with research at Edwards Air Force Base, Irvine, California.